The Use of Human Stem Cells to Promote Tissue Healing in Skin Burn Wound Injuries
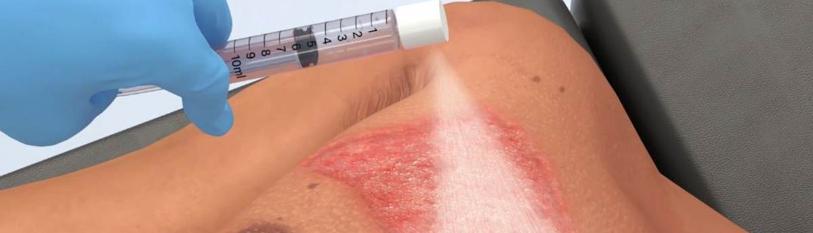
Taylor Petrucci and Vincent S. Gallicchio*
Department of Biological Sciences, College of Science, Clemson University, Clemson, South Carolina, USA
*Corresponding author: Vincent S Gallicchio, Department of Biological Sciences, College of Science, Clemson University, Clemson, South Carolina, USA
Citation: Petrucci T, Gallicchio VS. (2021) The Use of Human Stem Cells to Promote Tissue Healing in Skin Burn Wound Injuries. J Stem Cell Res. 2(1):1-14.
Received: December 17, 2020, | Published: January 04, 2021
Copyright© 2021 genesis pub by Petrucci T, et al. CC BY-NC-ND 4.0 DEED. This is an open-access article distributed under the terms of the Creative Commons Attribution-Non Commercial-No Derivatives 4.0 International License., This allows others distribute, remix, tweak, and build upon the work, even commercially, as long as they credit the authors for the original creation.
DOI: https://doi.org/10.52793/JSCR.2021.2(1)-15
Abstract
Skin burn wounds can be very serious injuries with high-risk healing processes and leaving life-long effects, regardless of the mechanism of injury. Traditional methods of treatment such as skin grafts and early excision are unable completely regenerate the skin. Therefore, new treatment avenues are being explored; one of the most promising potential treatments is the use of stem cells to enhance skin repair. Although multiple stem cells have been evaluated, more research is necessary to determine which method is most successful for widespread clinical treatment. Currently, Adipose-derived stem cells (ASCs) show the most promise in preliminary research and current clinical trials, but mesenchymal stem cells (MSCs), bone marrow stem cells (BMSCs), and umbilical cord stem cells (UCMSCs) are also currently being experimented with. Finally, economic factors as well as technological boundaries must also be overcome in order for stem cell therapy to replace traditional clinical treatments of burn wounds.
Keywords
Stem cells; Burns; Skin wounds; Healing
Introduction
Stem cells are classified by their origin as either embryonic or adult stem cells according to the developmental stage. They are undifferentiated pluripotent cells that generate cells and tissues from either ecto-, meso- or endoderm layers [1]. Stem cells are available on reserve to repair damaged cells that release stress signals [2]. Embryonic and adult stem cells have the ability to differentiate into a variety of tissue types [3]. Sources such as bone marrow, umbilical cord blood, adipose tissue, skin and hair follicles have lent stem cells to studies measuring the acceleration of the healing process in burn wounds [3,4]. Additionally, stem cell biology has become a growing interest of many medical researchers because stem cells have the capacity to renew themselves as well as differentiate multiple cell types [5].
In the skin, most stem cells are located within the hair follicle bulge [6]. Stem cells repair a wound by giving rise to daughter skin cells, which migrate to the epidermis [7]. These stem cells do not normally supply cells to the epidermis, but only do so after epidermal injury [7]. Severe burns are a highly common soft tissue injury that cause extensive damage to the skin. Severe burns often result in unsatisfactory functional and cosmetic healing [8]. They are a devastating trauma which requires specialized, immediate care [9]. Stem cell therapy is an indicated treatment for severe burns because of the potential for enhanced wound healing, replaced skin, and perfect skin regeneration [9]. Burns also present systemic effects such as inflammation, hypermetabolism and immunosuppression which may be combatted with stem cell therapy [9].
Stem cell therapy is a viable treatment because it is efficient, high-quality therapeutic treatment for skin burns that carries a potential to address systemic effects of burn injury such as hypermetabolism and inflammation [9]. There are three major pathways through which stem cells accelerate burn wound healing: granulation tissue formation, collagen deposition, and by inducing neo-angiogenesis. They modulate the inflammatory response and reduce the risk of infection. They can regenerate skin appendages and halt the zone of stasis in acute burn injury [10].
Background
The skin is the body’s largest organ, and it is quite complex. It not only serves as a barrier to the outside world, but also functions in immune inflammatory processes. The skin’s structure includes the epidermis, or top layer, the dermis, or middle layer, and most internally, there is subcutaneous fat. The epidermis is the outermost layer of the skin and it acts as a wall to restrict access to the inside of the body. It consists primarily of keratinocytes with tight connections to form the stratum corneum, a critical piece of the barrier of the epidermis. The basement membrane separates the dermis from the epidermis. It is comprised of a thin layer of extracellular matrix proteins. The dermis is a more flexible and elastic layer than the epidermis and is also made up of extracellular matrix proteins. Finally, the subcutaneous fat layer consists of adipocytes, and also houses blood vessels, nerves, and lymphatic vessels [4].
Burn wounds include multiple mechanisms of injury which lead to similar results. Scalds are common type of thermal injury seen in children and the elderly population and they result from hot liquid spills or hot bathing water. These injuries typically cause superficial dermal burns. Another common mechanism of thermal injury is a flame burn which tends to cause deep dermal or full thickness burns. A smaller number of burns are caused via electrocution. This occurs when an electrical current travels through the body and the tissue through which it travels may face extensive damages. The depth of these wounds is determined by heat levels reached, and therefore relies on the voltage of the electrical current. This leads to a concern for cardiac abnormalities and requires extensive monitoring during treatment. Finally, chemical injuries result from accidental exposure to corrosive chemical agents and these wounds are typically deep [11].
There are three degrees of burns. First-degree burns are only injuries to the superficial epidermis. For these burns, only mild treatments are necessary, and risks are relatively low. Second-degree burns destroy the epidermis and damage the dermis; dermal damage can either be superficial or deep. There is a greater risk of hypertrophic scarring with these as well as the deeper third- and fourth degree burns which penetrate into subcutaneous fat and muscle, respectively [12] (Figure 1).
Figure 1: Depicts depth levels of different burn wound degrees [13].
Once a burn reaches 30% of total body surface area (TBSA), a systemic effect is seen in the body. Cardiovascular changes involve increased capillary permeability, which results in the loss of proteins and fluids to the interstitial space [11]. Fluid loss also occurs from the burn wound itself and these result in systemic hypotension [11]. Hypovolemia then leads to decreased perfusion and oxygen delivery [12]. Additionally, in severe burns, respiratory distress syndrome can occur because of bronchoconstriction [11]. The cell basal metabolic rate will also increase to as much as three times its normal rate which exacerbates the hypoperfusion and requires early medical intervention through enteral feeding to reduce catabolism [11]. Lastly included in the systemic response are immunological alterations including non-specific down regulation of the immune response which affects multiple cell pathways [11]. (Figure 2).
Figure 2: Depicts systemic changes that occur as the result of a burn injury 11].
Successful healing after a burn injury is related to burn size and depth, as well as confounding factors of the patient such as age, mechanism of injury, and other comorbidities [12]. In this case, success is measured by healing time and scar quality [14]. Furthermore, burns may present a progressive expansion of necrosis into the zone of stasis, which is an area of initially viable tissue that is not harmed until the burn progresses [15]. Success may also be found in preventing the expansion of this necrotic tissue and allowing for viable tissue to remain [15]. There are also three phases during wound healing: inflammation, proliferation, and remodeling; these phases may be referenced in (Table 1) 5]. These processes are highly regulated by growth factors, cytokines, chemokines and cells [5]. Proper wound treatment involves maintaining an appropriate environment, infection control, and debriding the tissue [5]. Every phase in wound healing is mediated by stem cell proliferation and signaling [16]. Therefore, impaired stem cell functioning leaves to chronic wounds, so stem cell-based treatments are a rational approach to chronic wound care [16].
Phase |
Characteristics |
Inflammatory |
Coagulation cascade stops bleeding, vasodilation, neutrophils increased to stop infection |
Proliferative |
Granulation tissue forms a loose network of collagen, fibronectin, and hyaluronic acid, angiogenesis, re-epithelization |
Remodeling |
Simultaneous scar formation and degradation, fading of inflammatory reaction, wound maturation |
Table 1: Names and compares the three phases of wound healing [5,17].
Descriptions of early excision of burn wounds began in 1510 AD with Ambroise Pare [18]. However, limitations of medical technology made excision of large burns impossible. More recently, Advancement of burn wound treatments has been significant in the last century. The late 1950s advanced the early excision technique with enhanced shock monitoring, however the technique was not widely accepted because of expected outcomes such as mortality, infection, excess blood loss, and extended healing times 18]. Treatment of burn wounds has remained a difficult medical problem to be solved.
Today, burn wound treatment begins with the removal of all necrotic tissue and debris [12]. Early excision coupled with autografting has improved burn patient outcomes dramatically over the past several decades [19]. Coverage of the burn wound helps to prevent fluid and protein loss, hypothermia, and the risk of infection [19]. Dressings that are biosynthetic, biological, dermal analogues, or derived from the patient have provided options beyond a simple meshed graft in recent years and allow for optimized healing (Table 2) [19].
EpiDex |
Autologous |
Keratinocyte-based |
Alloderm |
Acellular |
Human origin, Dermal Matrix |
GraftJacket |
Acellular |
Human origin, Tissue scaffold |
Integra |
Acellular |
Bovine/shark origin, Bilayer matrix |
Biobrane |
Acellular |
Biocomposite dressing, nylon fibers in silicone with collagen |
Dermagraft |
Cellular |
Bioabsorbable polyglactin mesh scaffold with human fibroblasts (neonatal origin) |
Epicel |
Cellular |
Keratinocyte- based cultured epidermal autograft |
Recell |
Cellular |
Autologous cell suspension of keratinocytes, fibroblasts, Langerhans cells and melanocytes, Sprayable after culture |
Table 2: Skin substitutes currently for sale on the commercial market [17].
Patients with severe burns often receive skin grafts, however donor skin and deficiency of eligible skin substitutions in severely burned patients hinders accuracy of treatment and prolongs hospital stays [18]. Patients are also often left with excess scarring and much less elasticity that normal skin [19]. Another new method involves application of antimicrobials to prevent wound infection [20]. Biofilms are a collection of microorganisms that delay wound healing by initiating a constant inflammatory response [20]. Finally, tissue engineering and regenerative medicine therapy have developed in an effort to overcome limitations of previously used treatment methods [20]. Stem Cell isolation techniques have enabled stem cells to be applied directly to injured areas in order to enhance wound closure [19]. Furthermore, stem cells included in multi-layer skin substitutes allow for complete repair and replacement of damaged tissue because they resemble normal skin more closely [19]. More specifically, dermal analogs and cultured epidermal autografts have been modified using stem cells to develop multipurpose products that replace both dermal and epidermal skin [21]. Traditional Bioengineered skin equivalents do not replace lost structures such as sebaceous and sweat glands, as well as hair follicles [22]. New techniques are also being used to further improve such engineered skin replacements. An example of these techniques is the addition of melanocytes to decrease hypopigmentation and achieve closer color matching [23]. Another example is the introduction of vascular endothelial growth factors and angiogenic cytokines to shorten healing time and prevent graft loss by counteracting the absence of a vascular plexus [24,25].
Stem cells allow for new opportunities in burn treatment as they have shown to improve the wound repair process both locally and systemically [19]. As a tool for healing burn wounds, stem cells show promise as they can be used to regenerate as well as accelerate re-epithelization [26]. These pluripotent cells can be clonally amplified in order to expedite epithelization and can also be transplanted without worries of rejection [19]. Stem cells lack immunogenic surface markers which gives them the privilege of avoiding rejection [27]. Stem cells are a valuable tool, but each type of stem cell must be evaluated for effectiveness in order to determine the best method of burn wound treatment (Table 3).
Cell |
Source |
Potency |
Advantages |
Mesenchymal stem cells |
Bone marrow, stroma, blood |
Pluripotent |
Pluripotent, clonogenic |
Umbilical cord |
Umbilical cord blood |
Pluripotent |
Pluripotent, non-immunogenic, clonogenic |
Resident progenitor cells |
Numerous tissues/organs |
Unipotent |
Accessible potential for trans-differentiation |
Adipose-derived stem cells |
Adipose tissue |
Multipotent |
Non-immunogenic, abundant supply, accessible, clonogenic |
Table 3: Depicts a review of stem cell nomenclature [28].
Discussion
Although Chunmeng et al., did not support the use of adult mesenchymal stem cells (MSCs), they found that systemic transplantation of dermis derived multipotent cells (DMC) promoted the healing of wounds [29]. However, DMC cultivation is widely unsuccessful and has a very low yield, so little further research has been performed to evaluate the effectiveness of DMCs in burn wound healing [22]. Another option would be to pursue treatment using hair follicle stem cells; however, these hair follicle stem cells are less common and have proved extremely difficult to populate ex-vivo [30].
Likewise, epidermal stem cells lack detailed research regarding their effectiveness in burn wound treatment (EPSCs). Llames et. al., Used a culture of EPSCs on a bed of fibroblasts embedded with a plasma matrix to enable restoration of both epidermal and dermal component [31]. Mice with third-degree burns were treated with EPSCs that were activated by human a-defensin-5 and it was found to both promote hair regeneration as well as accelerate wound healing [32]. The transplantation of LGR6-positive EPSCs isolated by fluorescence-activated cell sorting and administered by injection into the wound significantly promoted re-epithelization, hair growth, and angiogenesis [32]. Carrier substrates could create an optimized physiological environment that may improve the regenerative performance of EPSCs [33].
Adult mesenchymal stem cells (MSCs)
Most regenerative medicine research is focused on mesenchymal stem cells (MSCs) because they are derived mainly from bone marrow and adipose tissue [9]. CD34+ hematopoietic stem cells are the most widely studied and represent the only clinically approved stem cell [3].
MSCs can be derived from various tissues and have high differentiation potential [34]. MSCs are able to migrate to injured tissues, differentiate, and regulate tissue regeneration by the production of growth factors, cytokines, and chemokines [35]. Shumakov et al., performed experiments on 40 Wistar rats where MSCs were applied to wounds showing decreased cell infiltration of the wound [36]. Accelerated formation of new vessels and granulation tissue was noted in comparison with embryonic fibroblasts and controls, burn wounds with no transplanted cells [36]. Likewise, Abbas et al. found in their experimentation with Wistar rats that the vital tissue percentage within the zone of stasis was significantly higher in the stem cell group of rats [15]. Huo et al., found that MSCs have great potentials in the burn field [37]. However, the cell survival and outcome are also facing challenges from poor microenvironment of the burn wound – their struggle to survive in a burn wound environment is a potential draw-back of the use of MSCs [37].
Bone marrow stem cells (BMSCs)
Bone marrow derived stem cells (BMSC) were analyzed against embryonic fibroblasts by Shumakov et al. in the first use of BMSCs for burn wound healing research [14]. In 2004, Rasulov et al., were the first to report using BMSCs in humans [38]. They confirmed high tempo of wound regeneration in the presence of active neoangiogenesis [38]. Rasulov et al., on rats also showed the superiority of stem cells in burn wound healing [39]. Xue et al., explored BMSCs to improve burn wound healing in the skin of mouse models [29,40]. Wound surface healing was significantly accelerated as well as the number and density of new blood vessels increased [40]. These mice showed no corresponding tumor growth and they gained weight faster [29,40]. Ha et al. transfected BMSCs with adenovirus as a vector with hepatocyte growth factor (HGF) [29,40,41]. The group treated with the MSCs had more significant re-epidermal growth at a shorter interval, less type 1 collagen and a thicker epidermis [41]. Although BMSCs have been successful in recent trials, a search for effective stem cell therapies continues because of invasive methods of retrieval for BMSCs as well as their low cell yields [40,41].
Method |
Treatment |
Measurement Timeline |
Results |
Injected BMSCs into skin of scalded fore limbs of male mice |
0.7 x 106 viable cells in 60 μL of PBS (1 million cells)
|
Daily until wound was healed (covered by newly formed epithelium) |
Wound surface healing significantly accelerated |
Partial thickness skin burns on female rats |
BMSCs in adenovirus vector with HGF |
Days 3, 5, 7, 14, and 21 postburn |
BMSCs have positive effects on healing of burn wounds |
Umbilical cord mesenchymal stem cells (UCMSCs)
Umbilical cord stem cells (UCMSCs) are used as a source of mesenchymal stem cells [42]. They have endothelial progenitor cells, in addition to hematopoietic and non-haemopoietic stem cells. These UCMSCs can be transformed into skin, endothelium or bone [43]. Liu et al., used UCMSC on burns in rats and found expedited wound healing. Higher neo-angiogenesis, the formation of new blood vessels, was recorded [44]. Similarly, Li et al., used exosome from human UCMSCs to attenuate burn-induced excessive inflammation with 30% total body surface area (TBSA) burn wound on the dorsal side of rats [45]. A 30% TBSA full-thickness burn injury typically causes an excessive inflammatory response [45]. However, the administration of their hUCMSC-exosome reduced the number of white blood cells [45]. This reduction became especially clear after 24 hours, and Li et al., concluded that hUCMSC-exosome, in general, mildly suppressed inflammation after burn injury [45].
Zhou et al., created a human umbilical cord mesenchymal stem cell-conditioned medium in the form of a hydrogel, which could be topically applied to burn wounds [46]. Injured mice were treated with either conditioned or unconditioned hydrogel and compared with a control group [46]. Application of the UCMSC conditioned hydrogel shortened healing time, reduced area of inflammation, and enhanced reepithelization effectively in third-degree burned mice; however, effects were not found to be statistically significant 46]. Although topical application of the UCMSC allowed for ease of treatment, effects were not optimal and alternative treatments should be developed and evaluated [46].
Finally, Abo-Elkheir et al., compared BMSCs and UCMSCs with standard grafting and early excision of recent thermal full-thickness burned human patients 22]. Patients’ burn percentages ranged from 10% to 25% TBSA, and following treatment, the percentage of burn extent was significantly reduced in both UCMSC and BMSC groups compared to standard grafting and early excision groups [22]. Ultimately, however, no significant difference between UCMSC and BMSC groups was found and further research is required to determine if one is more effective than the other [22].
ASCs (adipose-derived stem cells)
Adipose-derived stem cells (ASCs) are pluripotent and capable of differentiating into all three germ layers [47]. These stem cells can be cultured to up to 1000 times their yield making them distinctly more attractive than MSCs [48]. Adipose tissue can be harvested by a minimally invasive procedure, which makes it a promising source of adult stem cells [49]. Fujiwara et al., used an ovine burn model and topical application of ASCs from non-injured healthy sheep 47]. They assessed wound healing at one- and two-weeks following application and treatment with ASCs was found to accelerate the patch graft growth compared to the control [47]. Topical application of ASCs significantly increased wound blood flow [47]. Expression of Vascular endothelial growth factor (VEGF) was significantly higher in the wounds treated with ASCs compared to those treated with placebo [47]. Foubert et al., compared local injection and topical application of ASCs [50]. They created full-thickness thermal burns on 10 Gottingen minipigs and either sprayed ADSCs onto the wound surface (0.25 × 106 viable cells/cm2) or performed multiple injections into or surrounding the wound bed [50]. No significant difference between delivery approaches was found, and they concluded that the spray system did not cause loss of cell number or viability and is recommended because less invasive [50]. Necrosis is a common complication in skin graft therapy [51]. Subsequently, Zografou et. al. measured the effectiveness of ASCs in the mitigation of necrosis after skin graft procedures on rats [51]. ASCs were isolated, cultured, and labelled with fluorescent dye and injected beneath a full-thickness skin graft on the dorsal side of ten rats [51]. The grafts were analyzed by assessing the Collagen’s framework, and the mean area of graft necrosis was significantly less in the injected group than control group [52]. They concluded that the autologous transplantation increased full-thickness skin graft survival via two pathways: in situ differentiation of ASCs into endothelial cells as well as increase secretion by ASCs of growth factors such as VEGF and TGFb3 which enhance angiogenesis and wound healing [51]. Finally, Zhou et al. isolated ASCs of Sprague-Dawley rats and labeled them with green fluorescent protein 53]. Previous success with ASCs in other research allowed Zhou et al. to compare different injection patterns of ASC therapy [53]. They concluded that multiple injections of the ASCs significantly accelerated the wound healing process and also efficiently participated in angiogenesis [53] (Table 4).
Method |
Treatment |
Measurement Timeline |
Results |
Topical application of ASCs to ovine burn model |
3.5 x 106 viable cells/cm2 |
One- and two-weeks post injury |
ASCs significantly increased growth & blood flow |
Injection and topical application (spray on) of ASCs on porcine model |
0.25 x 106 viable cells/cm2 |
days 7, 12, 16, 21, and 28 posttreatment |
No significant difference between treatment methods was found |
ASCs injected under full-thickness skin grafts in rats |
1 x 106 viable cells/cm2 |
One-week post injection |
Mean area of graft necrosis was significantly less in injected group than control group |
ASCs injected beneath 2-cm2 burn wound on dorsal skin of rat |
2 x 106 viable cells/cm2 injected either 1 or 3 times |
Digital images of wound area taken pre- and posttreatment and throughout healing |
Multiple injections increased healing and promoted angiogenesis |
Table 4: Compares animal studies using ASCs in a burn wound model.
Conclusion
When comparing each type of stem cell for potential success in burn wound treatment, adipose-derived stem cells (ASCs) seem to possess the least limitations for clinical applications [54]. These ASC’s stem cells are considered the “holy-grail” in regenerative medicine as they are widely available, easily obtainable and propagated, have pluripotent potential and promote wound healing [55]. As compared with BMSCs, ASCs are easier to access, and they yield higher amounts of stem cells when isolated [52]. BMSCs carry several disadvantages such as a painful isolation procedure, low cell yields, and the need for general anesthesia [52]. As far as properties of self-renewal and multipotential differentiation, both BMSCs and ASCs were shown to be similar, therefore making ASCs an attractive substitute for BMSCs [52]. When compared to BMSCs, UCMSCs were not significantly different, although both improved healing compared to traditional treatment methods [22].
As important as it is to identify the most effective stem cell therapy for burn wound treatment, the work will not stop there. Once successful stem cells are identified, work must be done to determine the best method of application and if additional treatments should be performed on the cells prior to application. For example, Imam and Rizk found success when using erythropoietin pre-treated stem cells [56]. Ramhormozi et al. also found success through the use of combination therapy utilizing BMSCs and simvastatin with increased wound closure and decreased overall healing times [57]. Thus, co-cultures with other cell types as well as other various pretreatments using chemicals or cell media should be explored in greater detail [58].
Economic barriers must also be overcome in order to adopt stem cell therapies for skin regeneration [16]. Costs of cell-based therapies are frontloaded, which delays return on investment [16]. Additionally, the technology to standardize and mass-produce such treatments is not yet available, so considerable funding must also be directed into the development of this large-scale infrastructure [16].
Currently, a search of clinical trials.gov including keywords “burn” and “stem cell” yielded 25 active clinical trials in the United States that are focused on these treatments [59]. Of these trials, seven are currently examining the success of Adipose-derived stem cells (ASCs) in burn wound treatment: NCT03686449, NCT03113747, NCT02779205, NCT03183648, NCT03183622, NCT02619851, NCT02394873 [59]. A summary of these trials can be found in Table 5. Each trial represents progress in the research of stem cell therapies for burn wounds. In particular, trial NCT03183648 is a phase 2 trial measuring the safety and tolerability of ALLO-ASC-DFU, a hydrogel sheet containing allogenic ASCs [59]. The ease of application of a hydrogel sheet as a wound dressing makes this method of delivery a favorable approach for both patients and practitioners [59] (Table 5).
Trial Identifier |
Study Title |
Conditions |
Interventions |
Phase |
NCT03686449 |
Autologous Keratinocyte Suspension Versus Adipose-Derived Stem Cell-Keratinocyte Suspension for Post-Burn Raw Area
|
Burn with Full-Thickness Skin Loss |
|
N/A |
NCT03113747 |
Allogenic ADSCs and Platelet-Poor Plasma Fibrin Hydrogel to Treat the Patients with Burn Wounds (ADSCs-BWs) |
Second- or Third-degree Burns |
|
Phase 1 Phase 2 |
NCT02779205 |
Child’s Adipose Cells: Capacity of Tissue Regeneration |
Burns |
|
N/A |
NCT03183648 |
A Follow-Up Study to Evaluate the Safety of ALLO-ASC-DFU in ALLO-ASC-BI-201 Clinical Trial |
Burn |
|
Not Listed |
NCT03183622 |
A Follow-Up Study to Evaluate the Safety of ALLO-ASC-DFU in ALLO-ASC-BI-101 Clinical Trial |
Burn |
|
Not Listed |
NCT02619851 |
A clinical Trial to Evaluate the Safety and Efficacy of ALLO-ASC-DFU for Second Deep Degree Burn Injury Subjects |
Burn Injury |
|
Phase 2 |
NCT02394873 |
A Study to Evaluate the Safety of ALLO-ASC-DFY in the Subjects with Deep Second-degree Burn Wound |
Burn |
|
Phase 1 |
Table 5: Summary of current clinical trials involving the use of ASCs [59].
Although the outlook of these ASC trials is promising, many therapies are still in early stages of development and remain in small animal models [60]. A devastating morbidity is associated with burn wound healing as poor outcomes may lead to lifelong disability and severe scarring [60]. There is a profound potential impact of stem cell therapy to facilitate wound healing and dramatically improve the lives of trauma and burn victims.
References
1. Lin G, Xu RH. (2010) Progresses and challenges in optimization of human pluripotent stem cell culture. Curr Stem Cell Res Ther. 5(3):207-14.
2. M Witkowska-Zimny M, Wrobel E. (2011) Perinatal sources of mesenchymal stem cells: Wharton’s jelly, amnion and chorion. Cell Mol Biol Lett. 16(3):493-514.
3. Branski LK, Gauglitz GG, Herndon DN, Jeschke MG. (2009) A review of gene and stem cell therapy in cutaneous wound healing, Burns. 35(2):171-180.
4. Richmond JM, Harris JE. (2014) Immunology and Skin in Health and Disease. Cold Spring Harb Perspect Med. 4(12):a015339.
5. L Su, J Zheng, Y Wang, W Zhang, D Hu. (1982) Biomedicine & pharmacotherapy, Biomedicine & Pharmacotherapy. 117 (1982) 109191.
6. Taylor G, Lehrer MS, Jensen PJ, Sun TT, Lavker RM. (2000) Involvement of follicular stem cells in forming not only the follicle but also the epidermis. Cell. 102(4):451-61.
7. Ito M, Liu Y, Yang Z, Nguyen J, Liang F, et al. (2005) Stem cells in the hair follicle bulge contribute to wound repair but not to homeostasis of the epidermis. Nat Med. 11(12):1351-4.
8. Ahmadi AR, Chicco M, Huang J, Qi L, Burdick J, et al. (2019) Stem cells in burn wound healing: A systematic review of the literature. Burns. 45(5):1014-23.
9. Anna Arno, Smith AH, Blit PH, Shehab MA, Gauglitz GG, et al. (2011) Stem cell therapy: a new treatment for burns? J Pharm. 4(10):1355-80.
10. Francis E, Kearney L, Clover J. (2019) The effects of stem cells on burn wounds: a review. IJBT. 9(1):1.
11.Hettiaratchy S, Dziewulski P. (2004) Pathophysiology and types of burns. Bmj. 328(7453):1427-9.
12. Kao CC, Garner WL. Acute burns. (2000) Plast Reconstr Surg. 105(7):2482-92.
13. Church D, Elsayed S, Reid O, Winston B, Lindsay R. (2006) Burn wound infections. Clinic Microbiol Rev. 19(2):403-34.
14. Ghieh F, Jurjus R, Ibrahim A, Geagea AG, Daouk H, et al. (2015) The Use of Stem Cells in Burn Wound Healing: A Review. BioMed Res Int. Pp. 684084.
15. Abbas OL, Ozatik O, Gonen ZB, Ogut S, Entok E, et al. (2018) Prevention of Burn Wound Progression by Mesenchymal Stem Cell Transplantation: Deeper Insights Into Underlying Mechanisms. Ann Plast Surg. 81(6):715-24.
16. Coalson E, Bishop E, Liu W, Feng Y, Spezia M, et al. (2019) Stem cell therapy for chronic skin wounds in the era of personalized medicine: From bench to bedside. Genes & diseases. 6(4):342-58.
17. Rowan MP, Cancio LC, Elster EA, Burmeister DM, Rose LF, et al. (2015) Burn wound healing and treatment: review and advancements. Crit care. 19(1):243.
18. Liu HF, Zhang F, Lineaweaver WC. (2017) History and Advancement of Burn Treatments. Ann Plast Surg. 78(2):S2-8.
19. Jeschke MG, Finnerty CC, Shahrokhi S, Branski LK, Dibildox M. (2013) ABA Organization and Delivery of Burn Care Committee. Wound coverage technologies in burn care: novel techniques. J Burn Care Res. 34(6):612-20.
20. Oryan A, Alemzadeh E, Moshiri A. (2017) Burn wound healing: present concepts, treatment strategies and future directions. J Wound Care. 26(1):5-19.
21. Boyce ST. (1995) Treatment of chronic wounds with cultured cells and biopolymers. Wounds. 7:24-9.
22. Abo-Elkheir W, Hamza F, Elmofty AM, Emam A, Abdl-Moktader M, et al. (2017) Role of cord blood and bone marrow mesenchymal stem cells in recent deep burn: a case-control prospective study. AJSC. 6(3):23.
23. Swope VB, Supp AP, Cornelius JR, Babcock GF, Boyce ST. (1997) Regulation of pigmentation in cultured skin substitutes by cytometric sorting of melanocytes and keratinocytes. JID. 109(3):289-95.
24. Supp DM, Boyce ST. (2002) Overexpression of vascular endothelial growth factor accelerates early vascularization and improves healing of genetically modified cultured skin substitutes. J Burn Care Rehabil. 23(1):10-20.
25. Supp DM, Supp AP, Boyce ST, Bell SM. (2000) Enhanced vascularization of cultured skin substitutes genetically modified to overexpress vascular endothelial growth factor. JID. 114(1):5-13.
26. Wu Y, Chen L, Scott PG, Tredget EE. (2007) Mesenchymal Stem Cells Enhance Wound Healing Through Differentiation and Angiogenesis. Stem Cells. 25(10):2648-59.
27. Burd A, Ahmed K, Lam S, Ayyappan T, Huang L. (2007) Stem cell strategies in burns care. Burns. 33(3):282-91.
28. Butler KL, Goverman J, Ma H, Fischman A, Yu YM, et al. (2010) Stem cells and burns: review and therapeutic implications. J Burn Care Res. 31(6):874-81.
29. Chunmeng S, Tianmin C, Yongping S, Xinze R, Yue M, et al. (2004) Effects of dermal multipotent cell transplantation on skin wound healing. J Surg Res. 121(1):13-9.
30. Lu W, Zhang YJ, Jin Y. (2009) Potential of stem cells for skin regeneration following burns. Expert Rev Dermatol. 4(2):97-9.
31. Llames SG, Del Rio M, Larcher F, García E, García M, et al. (2004) Human plasma as a dermal scaffold for the generation of a completely autologous bioengineered skin. Transplantation. 77(3):350-5.
32. Lough D, Dai H, Yang M, Reichensperger J, Cox L, et al. (2013) Stimulation of the follicular bulge LGR5+ and LGR6+ stem cells with the gut-derived human alpha defensin 5 results in decreased bacterial presence, enhanced wound healing, and hair growth from tissues devoid of adnexal structures. Plast Reconstr Surg. 132(5):1159-71.
33. Yang R, Liu F, Wang J, Chen X, Xie J, et al. (2019) Epidermal stem cells in wound healing and their clinical applications. Curr Stem Cell Res Ther. 10(1):229.
34. Shpichka A, Butnaru D, Bezrukov EA, Sukhanov RB, Atala A, et al. (2019) Skin tissue regeneration for burn injury. Stem Cell Res Ther. 10.
35. Burdon TJ, Paul A, Noiseux N, Prakash S, Shum-Tim D. (2011) Bone marrow stem cell derived paracrine factors for regenerative medicine: current perspectives and therapeutic potential. BMA. 2011.
36. Shumakov VI, Onishchenko NA, Rasulov MF, Krasheninnikov ME, Zaidenov VA. (2003) Mesenchymal bone marrow stem cells more effectively stimulate regeneration of deep burn wounds than embryonic fibroblasts. Bull Exp Biol Med. 136(2):192-5.
37. Huo Y, Duan H, Liu L, Hu Q, Chai J. (2013) Research progress of mesenchymal stem cells in burn wound repair. Zhongguo xiu fu chong jian wai ke za zhi= Zhongguo xiufu chongjian waike zazhi= Chinese journal of reparative and reconstructive surgery. 27(5):571-4.
38. Rasulov MF, Vasil’Chenkov AV, Onishchenko NA, Krasheninnikov ME, Kravchenko VI, et al. (2005) First experience in the use of bone marrow mesenchymal stem cells for the treatment of a patient with deep skin burns. Bull Exp Biol Med. 139(1):141-4.
39. Rasulov MF, Vasilenko VT, Zaidenov VA, Onishchenko NA. (2006) Cell transplantation inhibits inflammatory reaction and stimulates repair processes in burn wound. Bull Exp Biol Med. 142(1):112-5.
40. Xue L, Xu YB, Xie JL, Tang JM, Shu B, et al. (2013) Effects of human bone marrow mesenchymal stem cells on burn injury healing in a mouse model. Int J Clin Exp Pathol. 6(7):1327.
41. HA XQ, LÜ TD, Ling HU, Fang D. (2010) Dong, Effects of mesenchymal stem cells transfected with human hepatocyte growth factor gene on healing of burn wounds. CJT. 13(6):349-55.
42. Forraz N, McGuckin CP. (2011) The umbilical cord: a rich and ethical stem cell source to advance regenerative medicine. Cell Prolif. 44:60-9.
43. Harris DT. (2009) Non‐haematological uses of cord blood stem cells. Br J Haematol. 147(2):177-84.
44. Liu L, Yu Y, Hou Y, Chai J, Duan H, et al. (2014) Human umbilical cord mesenchymal stem cells transplantation promotes cutaneous wound healing of severe burned rats. PloS one. 9(2):e88348.
45. Li X, Liu L, Yang J, Yu Y, Chai J, et al. (2016) Exosome derived from human umbilical cord mesenchymal stem cell mediates MiR-181c attenuating burn-induced excessive inflammation. EBioMedicine. 8:72-82.
46. P Zhou, X Li, B Zhang, Q Shi, D Li, et al. (2019) A Human Umbilical Cord Mesenchymal Stem Cell-Conditioned Medium/Chitosan/Collagen/β-Glycerophosphate Thermosensitive Hydrogel Promotes Burn Injury Healing in Mice. Biomed Res Int. 2019: 5768285.
47. O Fujiwara, A Prasai, D Perez-Bello, A El Ayadi, IY Petrov, et al. (2020) Adipose-derived stem cells improve grafted burn wound healing by promoting wound bed blood flow. Burns Trauma. 8:2020.
48. T Utsunomiya, M Shimada, S Imura, Y Morine, T Ikemoto, et al. (2011) Human adipose-derived stem cells: potential clinical applications in surgery. Surgery Today. 41(2011): 18-23.
49. M Witkowska-Zimny, K Walenko. (2011) Stem cells from adipose tissue, Cellular & Molecular Biology Letters. 16(2011):236-257.
50. P Foubert, AD Gonzalez, S Teodosescu, F Berard, M Doyle-Eisele, et al. (2015) Adipose-Derived Regenerative Cell Therapy for Burn Wound Healing: A Comparison of Two Delivery Methods. Adv in Wound Care. 5(7):288–298
51. A Zografou, C Tsigris, O Papadopoulos, N Kavantzas, E Patsouris, et al. (2011) Improvement of skin-graft survival after autologous transplantation of adipose-derived stem cells in rats. J Plast Reconstr Aesthet Surg. 64(12):1647-56.
52. AS Klar, J Zimoch, T Biedermann. (2017) Skin Tissue Engineering: Application of Adipose-Derived Stem Cells. Biomed Res Int. 2017:9747010
53. X Zhou, K Ning, B Ling, X Chen, H Cheng, et al. (2019) Multiple Injections of Autologous Adipose-Derived Stem Cells Accelerate the Burn Wound Healing Process and Promote Blood Vessel Regeneration in a Rat Model. Stem Cells Dev. 28(21):1463-1472.
54. A Hassanshahi, M Hassanshahi, S Khabbazi, Z Hosseini-Khah, Y Peymanfar, et al. (2019) Adipose-derived stem cells for wound healing. J Cellular Physiol. 234(2019):7903-7914.
55. L Casteilla, V Planat-Benard, P Laharrague, B Cousin. (2011) Adipose-derived stromal cells: Their identity and uses in clinical trials, an update. World J Stem Cells. 3(2011):25-33.
56. RA Imam, A Rizk. (2019) Efficacy of erythropoietin-pretreated mesenchymal stem cells in murine burn wound healing: possible in vivo trans-differentiation into keratinocytes. Folia Morphol (Warsz). 78 (2019):798-808.
57. P Ramhormozi, J Mohajer Ansari, S Simorgh, M Nobakht. (2020) Bone Marrow-Derived Mesenchymal Stem Cells Combined With Simvastatin Accelerates Burn Wound Healing by Activation of the Akt/mTOR Pathway, J Burn Care Res. 41(5):1069-1078.
58. Kucharzewski, E Rojczyk, K Wilemska-Kucharzewska, R Wilk, J Hudecki, et al. (2019) Novel trends in application of stem cells in skin wound healing, Eur J Pharmacol. 843:307-315.
60. Pati S, Pilia M. Grimsley JM, Karanikas AT. (2015) Cellular Therapies in Trauma and Critical Care Medicine: Forging New Frontiers. Shock. 44(6):505-523.