The Gut Microbiome and its Impact on Neurological Health: A Systematic Review
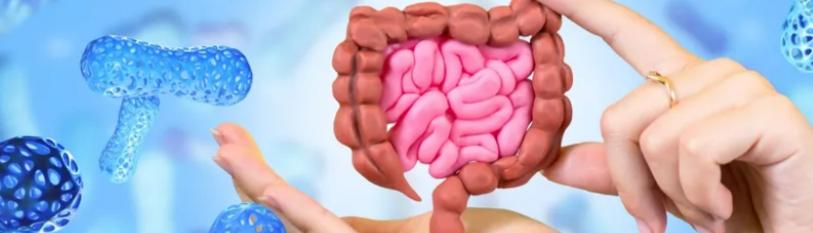
Karthik Chintharala1* , Salwa Asif2, Niharika Reddy3, Binay Kumar Panjiyar4 and Meghana Adusumilli1
1NRI Medical College & General Hospital-Guntur
2Department of Medicine, Faculty of Medicine, Tbilisi State Medical University
3Kasturba Medical College, Manipal
4GSCRT PGME Harvard Medical School, Research Fellow, Texas Tech University Health Sciences Center
*Corresponding author: Karthik Chintharala, NRI Medical College & General Hospital-Guntur
Citation: Chintharala K, Asif S, Reddy N, Panjiyar BK, Adusumilli M. The Gut Microbiome and its Impact on Neurological Health: A Systematic Review. J Neurol Sci Res. 4(1):1-19.
Received: April 30, 2024 | Published: May 15, 2024
Copyright©️ 2024 genesis pub by Chintharala K, et al.CC BY-NC-ND 4.0 DEED. This is an open-access article distributed under the terms of the Creative Commons Attribution-Non-Commercial-No Derivatives 4.0 International License. This allows others distribute, remix, tweak, and build upon the work, even commercially, as long as they credit the authors for the original creation.
Abstract
In recent years, neurological health has emerged as a leading global concern. Scientific studies have demonstrated that the gut microbiota plays a crucial role in this. Researchers now understand that the microbiota-gut-brain axis is critical for the formation and function of neurons and the pathogenesis of numerous neurological diseases. Thus, the significance of the gut-brain axis, mitochondrial and microglial dysbiosis, and their connection to neurological disorders is underscored by this systematic review. This paper further explains the bidirectional communication of signals between the gut to the brain, facilitated by mechanisms such as the vagus nerve, neurotransmitter formation, and the influence of short-chain fatty acids.
Keywords
Gut-Brain axis; Gut microbiome; Neurology; Gut microbiota
Introduction
In the human body, trillions of microbes reside that are assumed to influence and regulate the physiology of the host. The stomach and intestines (gastrointestinal tract or GIT) are where most microbes live, often called as gut microbiota. The GM is comprised of four major (Bacteroidetes, Firmicutes, Proteobacteria, and Actinobacteria) and two minor phyla (Verrucomicrobia and Fusobacteria) [1].
Good gut bacteria exhibit several beneficial effects on the host in a healthy state, but studies show that a disease state can lead to the development of various neurological disorders [1].
The maturation and development of the human central nervous system (CNS) is regulated by both intrinsic and extrinsic factors [2]. It has been found that the gut microbiota plays an important role in several physiological processes, such as the maintenance of homeostasis, immunomodulation, and regulation of the central nervous system (CNS) and enteric nervous system (ENS). Dysbiosis of the gut microbial communities is particularly associated with a variety of neurological disorders such as Parkinson’s disease (PD), Alzheimer’s disease (AD), multiple sclerosis (MS), autism spectrum disorder (ASD), and Major depressive disorder (MDD) [4]. Therefore, this systematic review provides insight into the involvement of GM and its impact on neurological health by analyzing the relationship between the GB axis and neurological abnormalities (Figure 1).
Figure 1: Shows a two-way connection between the enteric system and the brain in both healthy and sick individuals, Illustrated by Asif S.
The relationship between gut and CNS is complex as well as bidirectional anatomically also labelled as GB axis cross-talking each other in both health and disease contexts [1, 16]. With the help of this crosstalk, gut sensory visceral signals are allowed to travel through the vagus nerve so it may regulate the reflex and mind/mood changes by impacting the CNS, which in turn will allow the brain to lead the signals so it gut physiology and other functions can be modulated.
The GM also communicates with the brain through the vagus nerve, impacting gut motility and pain perception [1]. Furthermore, the microbiota's production of neurotransmitters influences the activity of the ENS [1]. It is critical to comprehend the mechanisms by which GM impacts cognitive function. To illustrate the point, specific bacterial byproducts, like SCFAs, influence the maturation of specific T cells and the production of different cytokines. Aryl hydrocarbon receptors can be activated by some bacteria in the gut, which astrocytes can subsequently use to produce anti-inflammatory effects [5]. Lipopolysaccharides (LPS) can cause neuroinflammation after migrating from the stomach to the brain, further demonstrating the connection between the two systems. The amygdala is a site of neurogenesis, and alterations in the gut microbiota can impact the integrity of the blood-brain barrier [6]. The vagus nerve plays a crucial role in this intricate process, whether it's through direct connections or enteric cells that are related to hormones and endocrine systems [3]. Notably, metabolites like glutamate and serotonin, influenced by the GM, exert physiological effects in both the gut and the brain [5].
The GB axis relies on a network of afferent and efferent neurons, encompassing various pathways such as the ENS, autonomic nervous system (ANS), sympatho-adrenal axis, hypothalamic-pituitary-adrenal (HPA) axis, and descending monoaminergic pathways [3]. This network integrates as well as monitors gut functions, connecting both cognitive and emotional centres in the brain with intestinal functions, including immune responses,entero-endocrine signalling, intestinal permeability and enteric reflexes[7]. The influence of GM on microglia and neurogenesis within the hippocampus is also a subject of intense research [4]. Dysfunctions in microglia have been linked to various neurodevelopmental and neurodegenerative diseases, with SCFAs, immune cells, and the vagus nerve playing integral roles in this complex interplay [7].
Furthermore, the intricate interplay between mitochondria and the GM has profound implications for overall health. Interestingly, mitochondria produce reactive oxygen species (ROS) that can have beneficial effects on specific cells. The GM also plays an active role in this interplay, with commensal bacteria releasing peptides that activate ROS in gut epithelial cells, aiding in the maintenance of the gut barrier and triggering anti-inflammatory responses. Gut dysbiosis, oxidative stress, and neuroinflammation significantly contribute to various neurodegenerative and autoimmune diseases [8]. In addition, recent studies have shown that GM has a major impact on the side effects and efficacy of several medications, including psychotropics. Mood and personality can be impacted by genetically modified substances, which encompass both antibiotics and non-antibiotic medications [9]. Polypharmacy, the use of multiple drugs simultaneously, has been associated with a decline in microbial diversity. Medications for depressive symptoms, proton pump inhibitors, and antipsychotics are examples of drugs that fall within this category. The action of some psychiatric medications is impeded by specific bacterial strains [10]. As a result, there are treatments and preventative methods that, with a better understanding of these intricate relationships, could be effective.
Methods
Neuroscience clinical studies involving the gut microbiota are the subject of this review. The review not only complies with the guidelines for Meta-Analyses (PRISMA) for 2020 and Preferred Reporting Items for Systematic Review in (Figure 2) but exclusively relies on data acquired from published studies, thereby the need for ethical approval being eliminated.
Figure 2: Haddaway, N. R., Page, M. J., Pritchard, C. C., & McGuinness, L. A. (2022). PRISMA2020: An R package and Shiny app for producing PRISMA 2020-compliant flow diagrams, with interactivity for optimized digital transparency and Open Synthesis Campbell System.
Methodical Literature Review and Study
As part of our systematic research evaluation and study selection process, we ran comprehensive research for pertinent publications using PubMed, which includes Google Scholar and Frontline. We used PubMed to find studies that were mentioned in editorials, commentaries, and review articles. Nonetheless, we persisted in seeking out further publications to fulfil our inclusion criteria. Using predetermined criteria, such as the presence of a well-described clinical cohort and the application of gut microbiota to neuroscience, we separately evaluated papers for inclusion in the study. Disagreements were settled through conversation after a review by three individuals.
Inclusion and Exclusion Criteria
A specific criteria was established to include and exclude participants so our study goal could be achieved. As briefed in (Table 1).
Inclusion Criteria |
Exclusion Criteria |
Human studies and Animal studies. |
|
From 2013 to 2023 |
Only clinical data, no full text access |
English Text |
Non-English texts |
Gender: All |
|
Age: > 19 years of age |
Age:<=19 years |
Free papers |
Papers that needed to be purchased |
Free full texts |
Paid papers |
|
Studies involving clinical data other than neurological disorders |
Table 1: Showing the criteria adopted during the literature search process.
Search Strategy
The PICO framework—which stands for "population," "intervention," "condition," and "control" or "comparison"—was utilized for this extensive literature review. Searches were conducted in databases such as PubMed and Google Scholar, among other Medical Libraries using terms relating to gut microbiota, gut microbiome, and neurological disorders. A comprehensive search strategy was devised using the medical subject heading (MeSH) approach for PubMed and Google Scholar, as outlined in Table (2). Thirteen papers were finalized.
PubMed |
(((((((microbiome[gut microbiota on neurological health [Title/Abstract]) OR (gut microbiota on neurological disorders [Title/Abstract])) OR (gut microbiome on neurological disorders[Title/Abstract])) AND (("2013/01/01"[Date - Publication] : "2023/09/01"[Date - Publication]))) OR (gut microbiota[MeSH Terms])) OR (gastrointestinal MeSH Terms])) OR (neurological disorders[MeSH Terms])) OR (neuropsychiatric disorders[MeSH Terms]) |
29,08,930 |
|
Filter Applied: Clinical trials, meta-analyses, randomized controlled trials, systematic reviews, and associated data over the last decade are all available for free in this abstract. English, All people, Person: 19 and up |
|
|
Papers deleted by finding duplicates and irrelevant articles |
11,062 |
|
Selected papers |
10 |
Google Scholar |
gut microbiota on neurological health or gut microbiota on neurological disorders or gut microbiome on neurological disorders |
61,000 |
|
Time range 2013-2023 |
18,500 |
|
from 1-10 pages of Google scholar |
105 |
|
Selected papers |
3 |
Table 2: Details the search process, including the keywords and engines used, as well as the total number of results shown.
Quality Appraisal Tools
To ensure the articles we selected were of high quality, we utilized a range of assessment tools. Utilizing the PRISMA protocol and the Cochrane method for bias evaluation for controlled clinical trials, we carried out our comprehensive Meta-analyses as well as reviews. Clinical trials that did not use random assignment were evaluated using the Newcastle-Ottawa methodology. We checked the thoroughness of qualitative studies using the Critical Appraisal Standards Program (CASP) rubric. We assessed the article's quality using the SANRA scale, so any room for interpretation in the classification could be avoided as indicated in (Table 3).
Quality Appraisal Tools Used |
Type of Studies |
Cochrane Bias Tool Assessment |
Randomized Control Trials |
Newcastle-Ottawa Tool |
Non-RCT and Observational Studies |
PRISMA Checklist |
Systematic Review |
AMSTAR |
Systematic Review |
Table 3: Showing quality appraisal tools used.
Results
After searching through two selected databases, PubMed and Google Scholar, we extracted 2,969,930 articles. Following a rigorous evaluation, 2,940,361 publications were excluded based on specific criteria. 28,407 papers out of the remaining 29,569 were not chosen due to them having unsatisfactory titles, abstracts or duplicates. 1,162 papers were thoroughly examined whereas 1,149 were excluded as the inclusion criteria were not met. Lastly, the remaining 13 papers were run through a detailed quality check all of which not only included in our final systematic review but also met our criteria. The (Table 4). below provides a detailed description of each.
Country |
Study Design |
Database Used |
Conclusion |
|
Korea |
Database Highlights |
PubMed |
This article emphasizes the importance of metabolites generated from the gut as critical components that cross the blood-brain barrier, activate microglia, and trigger inflammatory immunological pathways once they reach the brain. |
|
Korea |
Review |
PubMed |
This article discusses how the MGB axis controls biological networks that govern brain development and function, and whether or not the MGB axis has a role in neurological illnesses associated with the immune system. |
|
USA |
Review |
PubMed |
This article delves into the idea that genetic modifications have a significant role in how the nervous system develops and functions, as well as in maintaining a healthy equilibrium between mental illness and wellness. |
|
Spain |
Systematic Review |
PubMed |
This systematic review explains the correlation between GM dysbiosis and neurological or neuropsychiatric disorders such depression, Alzheimer's disease, and Parkinson's disease. It examines the possible advantages of supplemental psychobiotics for certain diseases. |
|
Belgium |
Systematic Review |
PubMed |
Neurological diseases such as Alzheimer's, Parkinson's, MS, and ALS are associated with altered gut flora, according to this comprehensive research. |
|
Portugal |
Systematic Review |
PubMed |
In an effort to prove that dysbiosis affects CNS disorders or that diseases induce dysbiosis, this comprehensive review looked at how GM affected brain function. |
|
Iran |
Comprehensive Review |
PubMed |
This article gives a thorough synopsis of the possible role of those who are GM in the development of neurological disorders. |
|
Korea |
Review |
PubMed |
The effects of genetic modification (GM) on mitochondrial function and exercise performance, as well as their interaction, are covered in this article. |
|
Spain |
Review |
PubMed |
This article examines neurological disorders where changes in the GM have been reported, as well as the function of the GM in the GB axis. |
|
China |
Systematic Review |
Google Scholar |
Key regions highlighted by the research include the MGB nexus and neuroinflammation. |
|
Malaysia |
Systematic Review |
Google Scholar |
This research delves into the GB center and its impact on various elements of communication, including anatomy, metabolism, the immune system, and endocrine function. Immune functioning, mood, cognition, and mental wellness are impacted by this communication, which is facilitated by the ANS, HPA axis, and gastrointestinal nerves. |
|
USA |
Database Highlights |
PubMed |
This article explores how microglia in the brain are influenced by the GM, impacting neurological disorders. |
|
Table 4: Summary of the outcomes of the articles that were chosen. ALS stands for Amyotrophic Lateral Sclerosis, and CNS is an abbreviation for the Central Nervous System.
Discussion
There is growing evidence from clinical trials, epidemiological studies, and immunological investigations indicating that the microbiota in the intestines affects the gut-brain axis, which includes cognitive function, emotional control, neuromuscular tone, and hypothalamus-pituitary-adrenal axis regulation [19]. The autonomic nervous system, hypothalamic-pituitary-adrenal axis, and vagus nerve connect the brain to the gut, enabling the former to affect the latter's functions, such as the activity of functional immunological effector cells [19]. However, scientists are still trying to figure out how gut microbiota works, but they know that it affects the brain’s emotion and cognition centres and that changes in these areas' communication circuits are associated with gut microbiota variations [19]. As an example, there are now well-established connections between changes in the gut microbiota and functional gastrointestinal disturbances in several mood disorders, including depression, anxiety, and autism spectrum disorders [19]. Disruption of the blood-brain barrier, activation of microglial cell populations and astrocytes, and neuroinflammation can occur when microbiota in unhealthy states produce more inflammatory cytokines, lipopolysaccharides, and T and B cells while decreasing the quantity of short-chain fatty acids [1]. Therefore, there is mounting evidence that changes in the makeup of gut bacteria may impact cerebral processes and vice versa, as a result of the two-way link [21].
Based on the connections between gut microbiota and neuronal function as well as cerebral processes, experimental and clinical data point to the intestinal microbiota as an essential component of the gut-brain axis. The cells of the intestinal tract and the enteric nervous system are local targets of its effects, but it also has direct communication with the central nervous system via endocrinology and metabolic pathways linked to memory, anxiety, and stress [7]. Crucially, the vagus nerve is the primary modulatory constitutive channel that links the brain to the microbiota, and it is involved in this communication [22]. The gut-brain axis is modulated by microbiota, which in turn, affects gut motility and pain perception by regulating afferent sensory nerves and increasing their excitability by blocking calcium-dependent potassium channels [23].
Expanding on the vast influence of bacteria on the enteric nervous system, their effect extends to the production of neurotransmitters, including acetylcholine, serotonin, melatonin, histamine, and gamma-aminobutyric acid [24]. An effective form of catecholamines is also generated in the gut lumen by these microbial populations [25]. Key bacterial metabolites, including butyric acid, propionic acid and acetic acid, impact memory and learning processes, stimulate the parasympathetic nervous system and influence the gut-brain axis via their metabolic byproducts and short-chain fatty acids (SCFAs) [26], [27]. The intestinal immune system’s impacts on the gut microbiota's ability to regulate the activity of the enteric nervous system is discussed in (Figure 3).
Figure 3: Intestinal immune system impacts the gut microbiota's ability to regulate the activity of the enteric nervous system, Illustrated by Asif S.
The discharge of physiologically active chemicals from enteroendocrine cells may modulate the effect of dietary changes on behaviour on microbiota composition [19]. Examples of peptides that can activate the hypothalamus-pituitary-adrenal axis include galanin [19]. This can lead to an increase in the secretion of glucocorticoids from the cortex of the adrenals, cortisol from norepinephrine from the adrenal medulla and adrenocortical cells. Similarly, ghrelin is known to release cortisol and adrenocorticotropin hormone, and it is believed that it plays a role in regulating the hypothalamus-pituitary-adrenal axis reaction to stress and changes in diet and metabolism [28]. Moreover, recent molecular research highlights that gut microbiota affects the central nervous system and enteric nervous system [7]. It further explains how gut microbiota impacts mucosal immune activation, as well as neurogenic inflammatory processes that arise from micro-element deficiencies secondary to functional and morphological changes in the digestive tract [31]. These processes can further link with the discharge of physiologically active chemicals from enteroendocrine cells, affecting behavior, and triggering the hypothalamus-pituitary-adrenal axis [29]. Additionally, in intestinal-immune mediated disorders it is highlighted that proteases are up-regulated and also serve as end-stage effectors of enteric nervous damage and mucosal [30]. Gut dysbiosis often leads to the loss of gut barrier integrity and increased intestinal permeability[6]. This state promotes the development of many neurological disorders by facilitating the increased translocation of metabolites produced by gut bacteria and microbe-associated molecular patterns into regional lymphoid tissues [1]. Glutamate, an important component in brain and gut physiological reactions [32], is found in both food sources and gut microorganisms, capable of producing glutamine. Glutaminase catalyzes the deamination of glutamine and its subsequent synthesis from α-ketoglutarate, both produced by neurons in the central nervous system [33]. Also, the astrocytic cytosol is rich in glutamine, which is then transported into the extracellular fluid and absorbed by neurons for glutamate synthesis. This leads to neuronal excitability that in turn alters in a pathogenic way [34]. Further, it seems that glutamate transmits signals from the epithelial layer to the enteric nervous system, functioning as a sensory transporter [35]. The main mechanism by which glutamatergic neurotoxicity is amplified is by selective activation of N-methyl-D-aspartate receptors within synapses rather than those outside of them [36]. Hydroxy tryptamine (5-HT) is another important neurotransmitter made by the gut microbiota from tyrosine with the help of tyrosine hydroxylase 1 [37]. Its significance in normal digestive systems is shown by the fact that 5-HT receptor antagonists affect enteric nervous system functioning [63]. Mutations in dopamine hydroxylase 2, have been associated with problems affecting enteric nervous system development and gastrointestinal functions [63]. A possible link between 5-HT and disorders like depression may lie in the fact that altered 5-HT levels in enteric neurons impact colon motility and gut transit rates [63].
Gut metabolites, such as short-chain fatty acids, produced by gut bacteria also affect 5-HT synthesis [37]. By interacting with orphan receptors coupled with G proteins (GPCRs), short-chain fatty acids, mainly function as signalling molecules. Furthermore, they inhibit histone deacetylases (HDACs) and interact with Sensory receptor 78 (Olfr78) [38], [39]. The immunological, vagal, endocrine and humoral pathways are the four primary means by which short-chain fatty acids impact central nervous system functioning. To influence brain processes, they directly activate immune cells associated with the enteric nervous system and vagal neurons to increase the production of regulated cytokines and peptides produced from the gut [1]. In addition to metabolites, components of the gut microbiota can influence the activities of the central nervous system and the endocannabinoid system by activating toll-like receptors, expressed in several brain cells, including enteric neurons, and cutaneous afferent cells [17]. Specifically, microglial cells, can increase inflammatory cytokine expression in the central nervous system and the gut as a result of LPS/TLR4 signaling [40], affecting the survival of the neurons in the enteric nervous system [41]. The presence of lipopolysaccharide at the blood-brain barrier raises the possibility that, under normal circumstances, it enters the brain via a lipoprotein mechanism for transport [42]. The abnormal levels of lipopolysaccharide make their way to the brain via the bloodstream, where they interact with toll-like receptor 4, triggering a cascade of harmful signalling events that lead to neural inflammation along with various neurological problems [1]. TLR4 is involved in neuroprotection from experimental brain injury, activating vascular endothelial cells and microglia, and other key processes [43], [42]. The transcription factor, Krüppel-like factors 4 (KLF4) is implicated in neuroinflammation and is involved in LPS-induced activation of microglia as well [44]. Microglia are like guardians of the brain, monitoring neuroinflammation, synaptic remodelling, and neurogenesis [11]. Many neurodevelopmental and neurodegenerative disorders, including schizophrenia, autism spectrum disorder, diseases such as multiple sclerosis, Alzheimer's illness, amyotrophic lateral sclerosis, Parkinson's condition, major depressive disorder, and multiple sclerosis are linked to microglial activity [20][13]. Microglial dysfunction and microbial dysbiosis in neurological diseases are discussed in (Figure 4).
Figure 4: Microglial dysfunction and microbial dysbiosis in neurological diseases.
Although microglia mostly respond to signals within the brain, new evidence suggests that they are not autonomous cells; but rather receive information from the periphery, specifically the gastrointestinal tract [20], as explained in the Gut Microbiota-Microglia Nexus. The intestinal microbiota may regulate several processes that impact microglia maturation and function [62]. Firstly, short-chain fatty acids generated by the gut microbiota can control the function or development of microglia by making their way through the host's circulatory system and eventually crossing the blood-brain barrier [62]. Secondly, short-chain fatty acid signals produced in the gut flora may cause immune cells with SCFA-recognition receptors to migrate across the blood-brain barrier and into the brain [62]. Furthermore, vagus nerves may allow the gut microbiota to communicate directly with microglia located in the brain [62]. Before the development of SCFA-recognizing receptors, microglia can be influenced by other metabolites or microbe-associated molecular patterns produced by the microbes in the gut [62]. Lastly, gut flora generates metabolites or microbe-associated molecular patterns that can be recognized by peripheral macrophages, which then travel to the brain across the blood-brain barrier in response to signals from these molecules [62].
Through the production of immune-related chemicals in the brain, such as cytokines, the major histocompatibility complicated (MHC), and Toll-like receptors, the host microbiome affects both responses to infection and brain development [1]. Additionally, the finding of meningeal lymphatic arteries in the brain suggests a possible connection between the central nervous system and the peripheral nervous system, which could impact autoimmune processes [45]. Moreover, available research points out the crucial functions of microglia and lymphocytes in controlling central nervous system immunological defence, neuronal circuitry, and cognition [46]. Furthermore, the gut microbiome continues to shape brain immunity throughout life by influencing microglial cell maturation, which begins during early development and continues throughout adulthood [47].
Recent research also highlights the importance of the gut microbiota's interaction with mitochondria for human health. Although this interaction is complex and context-dependent, the gut microbiota may regulate mitochondrial inflammatory responses through short-chain fatty acids and other pathways [48]. Mitochondrial production of ATP and NADH depends on short-chain fatty acids, particularly butyrate. The microbiota procures bile acids, which influence energy metabolism and regulate mitochondria. Nevertheless, mitochondrial energy production can be severely impacted by infections and high-protein diets [48]. Also, variables such as PGC-1α affect mitochondrial activity and energy production during endurance exercise. When you work out, your mitochondria release more reactive oxygen and nitrogen species through mitochondrial leaks. Although these compounds promote healthy blood vessel function and muscular development, they have the potential to damage DNA and mitochondria, hastening the ageing process [49]. The degree to which one exercises affects the onset of acute inflammation. Exercising causes mitochondrial stress, which in turn raises reactive oxygen and nitrogen production, activating inflammasomes [15]. A defence strategy against pathogens can include reactive oxygen species generation in mitochondria; however, pathogenic bacteria can adapt to control this process [15]. Disruption of the gut environment and immune system responses can be caused by dysregulated mitochondrial functions [50], [51]. Endurance exercise can cause mitochondria to multiply, which could lead to the accumulation of mutations, impacting mitochondrial functioning and the composition of the gut microbiota [15]. Diseases such as multiple sclerosis can be influenced by the oxidation and genetic/epigenetic alterations that can occur in mitochondrial DNA [15]. While reactive oxygen species in the gut can trigger protective mechanisms at lower levels, too much of them can damage mitochondria and lead to diseases like cancer (which is often caused by inefficient mitophagy) [59]. On the other hand, reactive oxygen species produced during respiration in the mitochondria can have beneficial effects on some cells in the gut. Additionally, the gut-brain axis connects the gut to mitochondria and brain health; hence, gut microbiota metabolites and dietary components can interfere with mitochondrial metabolism, leading to inflammation and oxidative stress discussed in (Figure 5).
Figure 5: Health benefits linked to regular exercise and a healthy digestive system.
There is mounting evidence that the gut microbiome plays a major role in determining the efficacy and safety of many drugs, including psychotropics. Antidepressants like selective serotonin reuptake inhibitors and other non-antibiotic medications have been investigated for their potential antimicrobial effects, and results have indicated that they inhibit a variety of bacterial strains [60]. According to research, certain microbial populations can be altered and reduced when using psychiatric medicines like desipramine and tricyclic antidepressants. There is evidence that atypical antipsychotics reduce microbial diversity, especially in females [61]. Furthermore, previous studies suggest that a decrease in the durability of the digestive barrier, caused by an aberrant intestinal microbiota, increases the number of lipopolysaccharides accessing the body, triggers systemic inflammation, and enhances the stress response [52]. However, there are several ways in which probiotics might lessen the physiological stress of the hypothalamus-pituitary–adrenal system and protect the intestinal barrier from harm [53], [54],[18]. The vagus nerve and the hippocampus secrete chemicals including oxytocin and brain-derived neurotrophic factor. Additionally, probiotics from the bacteria, Lactobacillus rhamnosus can lower the amount of corticosterone in the blood and alleviate depression [55,56]. The effects of probiotics may vary from patient to patient. In healthy people, daily intake of L. Caseiprobiotic supplement may help reduce stress symptoms and improve the composition of gut microbiota, according to a study [57]. Another study highlighted that stress conditions and negative mood management improved in healthy people who consumed the strain for 30 days, alongside a diet that included plants such as L. Helveticus and B. Longum [58]. In 2017, Bamling et al., tested a novel combination of antidepressants that included probiotics, magnesium, a mineral, and SSRIs, to impact therapy-resistant depression (TRD). Positive outcomes included a marked improvement in individuals' depressed symptoms [20].
Psychobiotics, also called psychomicrobiotics, are a new kind of probiotics that have just come to light as a non-toxic treatment for a range of mental health issues. Some probiotics have been tested in clinical trials for their potential to alleviate anxiety and depression [12]. However, this strategy for tackling anxiety and depression needs further validation in clinical trials with randomized controls due to the variation in probiotic dosage, strain selection, and treatment durations in existing investigations [12].
Limitations and Recommendations
Although there is a growing body of evidence linking gut microbiota to neurological health, establishing causality is difficult, as much research is predominantly observational or correlative. Future studies should focus on conducting more controlled experiments and clinical trials to better elucidate causal relationships. Another limitation is the fact that many drugs affect the gut bacteria. Future studies need to find out what kind of bacteria are involved and how metabolites affect neurological function, potentially leading to the discovery of beneficial microbial strains and interventions. It's still unknown how things like food choices and lifestyle can influence the gut-brain connection. Understanding these factors can help create possible preventive measures by learning how they affect the gut bacteria and, in turn, neurological health.
Conclusion
This systematic review highlights the important role of gut microbiota in affecting brain function, and its possible effects on neurological disorders. The gut-brain axis can either make brain problems worse or better. Hence, this paper concludes that an imbalance in the gut's microbiota can cause brain inflammation, chemical imbalances, and microglial and mitochondrial impairment— all of which are common features in various neurological disorders. This suggests that the gut-brain axis, through infections, metabolites, immune communication, and microglial and mitochondrial dysbiosis, impacts neurophysiological function and cognition. Understanding these complicated connections helps find new ways of treatment approaches aimed at improving neurological health outcomes for people suffering from neurological diseases.
References
- Suganya K, Koo BS. Gut-Brain Axis: Role of Gut Microbiota on Neurological Disorders and How Probiotics/Prebiotics Beneficially Modulate Microbial and Immune Pathways to Improve Brain Functions. Int J Mol Sci. 2020 Oct 13;21(20):7551. doi: 10.3390/ijms21207551. PMID: 33066156; PMCID: PMC7589356.
- Ma, Q., Xing, C., Long, W. et al. Impact of microbiota on central nervous system and neurological diseases: the gut-brain axis. J Neuroinflammation 16, 53 (2019).
- Sorboni SG, Moghaddam HS, Jafarzadeh-Esfehani R, Soleimanpour S. A Comprehensive Review on the Role of the Gut Microbiome in Human Neurological Disorders. ClinMicrobiol Rev. 2022 Jan 19;35(1):e0033820. doi: 10.1128/CMR.00338-20. Epub 2022 Jan 5. PMID: 34985325; PMCID: PMC8729913.
- Mayer EA. Gut feelings: the emerging biology of gut-brain communication. Nat Rev Neurosci. 2011 Jul 13;12(8):453-66. doi: 10.1038/nrn3071. PMID: 21750565; PMCID: PMC3845678.
- Sittipo, P., Choi, J., Lee, S. et al. The function of gut microbiota in immune-related neurological disorders: a review. J Neuroinflammation 19, 154 (2022).
- Carabotti M, Scirocco A, Maselli MA, Severi C. The gut-brain axis: interactions between enteric microbiota, central and enteric nervous systems. Ann Gastroenterol. 2015 Apr-Jun;28(2):203-209. PMID: 25830558; PMCID: PMC4367209.).
- Kunst, C.; Schmid, S.; Michalski, M.; Tümen, D.; Buttenschön, J.; Müller, M.; Gülow, K. The Influence of Gut Microbiota on Oxidative Stress and the Immune System. Biomedicines 2023, 11, 1388.
- Cussotto S, Clarke G, Dinan TG, Cryan JF. Psychotropics and the Microbiome: a Chamber of Secrets…. Psychopharmacology (Berl). 2019 May;236(5):1411-1432. doi: 10.1007/s00213-019-5185-8. Epub 2019 Feb 26. PMID: 30806744; PMCID: PMC6598948.
- Ticinesi A, Milani C, Lauretani F, Nouvenne A, Mancabelli L, Lugli GA, Turroni F, Duranti S, Mangifesta M, Viappiani A, Ferrario C, Maggio M, Ventura M, Meschi T. Gut microbiota composition is associated with polypharmacy in elderly hospitalized patients. Sci Rep. 2017 Sep 11;7(1):11102. doi: 10.1038/s41598-017-10734-y. PMID: 28894183; PMCID: PMC5593887.
- Sharon G, Sampson TR, Geschwind DH, Mazmanian SK. The Central Nervous System and the Gut Microbiome. Cell. 2016 Nov 3;167(4):915-932. doi: 10.1016/j.cell.2016.10.027. PMID: 27814521; PMCID: PMC5127403.
- Barrio C, Arias-Sánchez S, Martín-Monzón I. The gut microbiota-brain axis, psychobiotics and its influence on brain and behavior: A systematic review. Psychoneuroendocrinology. 2022 Mar;137:105640. doi: 10.1016/j.psyneuen.2021.105640. Epub 2021 Dec 17. PMID: 34942539.
- Bonnechère B, Amin N, van Duijn C. What Are the Key Gut Microbiota Involved in Neurological Diseases? A Systematic Review. Int J Mol Sci. 2022 Nov 8;23(22):13665. doi: 10.3390/ijms232213665. PMID: 36430144; PMCID: PMC9696257
- Almeida C, Oliveira R, Soares R, Barata P. Influence of gut microbiota dysbiosis on brain function: a systematic review. Porto Biomed J. 2020 Mar 17;5(2):1-8. doi: 10.1097/j.pbj.0000000000000059. PMID: 33299942; PMCID: PMC7722401
- Imdad S, Lim W, Kim JH, Kang C. Intertwined Relationship of Mitochondrial Metabolism, Gut Microbiome and Exercise Potential. Int J Mol Sci. 2022 Feb 28;23(5):2679. doi: 10.3390/ijms23052679. PMID: 35269818; PMCID: PMC8910986.
- Castillo-Álvarez F, Marzo-Sola ME. Role of the gut microbiota in the development of various neurological diseases. Neurologia (Engl Ed). 2022 Jul-Aug;37(6):492-498. doi: 10.1016/j.nrleng.2019.03.026. Epub 2021 May 25. PMID: 35779869
- Han S, Cai L, Chen P and Kuang W (2023) A study of the correlation between stroke and gut microbiota over the last 20 years: a bibliometric analysis. Front. Microbiol. 14:1191758. doi: 10.3389/fmicb.2023.1191758.
- Siripaopradit Y, Chatsirisakul O, Ariyapaisalkul T. Unlocking the Potential of the Gut-Brain Axis for Alzheimer’s Disease Treatment through Probiotic Interventions: A Systematic Review. J Asian Med Stud Assoc [Internet]. 2023Jul.11 [cited 2023Sep.10];. Available from:
- Appleton J. The Gut-Brain Axis: Influence of Microbiota on Mood and Mental Health. Integr Med (Encinitas). 2018 Aug;17(4):28-32. PMID: 31043907; PMCID: PMC6469458.
- Reem Abdel-Hag, Johannes C.M. Schlachetzki, Christopher K. Glass, SarkisK.Mazmanian. "Microbiome Microglia Connections via the Gut-Brain Axis." Journal ofExperimental Medicine. January 2019: 216 (1): 41-59.
- Cryan JF, O'Riordan KJ, Sandhu K, Peterson V, Dinan TG. The gut microbiome in neurological disorders. Lancet Neurol. 2020 Feb;19(2):179-194. doi: 10.1016/S1474-4422(19)30356-4. Epub 2019 Nov 18. PMID: 31753762.
- Bravo JA, Forsythe P, Chew MV, Escaravage E, Savignac HM, Dinan TG, Bienenstock J, Cryan JF. Ingestion of Lactobacillus strain regulates emotional behavior and central GABA receptor expression in a mouse via the vagus nerve. Proc Natl AcadSci U S A. 2011 Sep 20;108(38):16050-5. doi: 10.1073/pnas.1102999108. Epub 2011 Aug 29. PMID: 21876150; PMCID: PMC3179073
- Kunze WA, Mao YK, Wang B, Huizinga JD, Ma X, Forsythe P, Bienenstock J. Lactobacillus reuteri enhances excitability of colonic AH neurons by inhibiting calcium-dependent potassium channel opening. J Cell Mol Med. 2009 Aug;13(8B):2261-2270. doi: 10.1111/j.1582-4934.2009.00686.x. Epub 2009 Feb 4. PMID: 19210574; PMCID: PMC6529958.
- Iyer LM, Aravind L, Coon SL, Klein DC, Koonin EV. Evolution of cell-cell signaling in animals: did late horizontal gene transfer from bacteria have a role? Trends Genet. 2004 Jul;20(7):292-9. doi: 10.1016/j.tig.2004.05.007. Erratum in: Trends Genet. 2005 Jan;21(1):36. PMID: 15219393.
- sano Y, Hiramoto T, Nishino R, Aiba Y, Kimura T, Yoshihara K, Koga Y, Sudo N. Critical role of gut microbiota in the production of biologically active, free catecholamines in the gut lumen of mice. Am J PhysiolGastrointest Liver Physiol. 2012 Dec 1;303(11):G1288-95. doi: 10.1152/ajpgi.00341.2012. Epub 2012 Oct 11. PMID: 23064760
- Kimura I, Inoue D, Maeda T, Hara T, Ichimura A, Miyauchi S, Kobayashi M, Hirasawa A, Tsujimoto G. Short-chain fatty acids and ketones directly regulate sympathetic nervous system via G protein-coupled receptor 41 (GPR41). Proc Natl AcadSci U S A. 2011 May 10;108(19):8030-5. doi: 10.1073/pnas.1016088108. Epub 2011 Apr 25. PMID: 21518883; PMCID: PMC3093469
- Grider JR, PilandBE. The peristaltic reflex induced by short-chain fatty acids is mediated by sequential release of 5-HT and neuronal CGRP but not BDNF. Am J PhysiolGastrointest Liver Physiol. 2007 Jan;292(1):G429-37. doi: 10.1152/ajpgi.00376.2006. Epub 2006 Sep 14. PMID: 16973914
- Giordano R, Pellegrino M, Picu A, Bonelli L, Balbo M, Berardelli R, Lanfranco F, Ghigo E, Arvat E. Neuroregulation of the hypothalamus-pituitary-adrenal (HPA) axis in humans: effects of GABA-, mineralocorticoid-, and GH-Secretagogue-receptor modulation. ScientificWorldJournal. 2006 Jan 17;6:1-11. doi: 10.1100/tsw.2006.09. PMID: 16432622; PMCID: PMC5917168.
- Saito T, Bunnett NW. Protease-activated receptors: regulation of neuronal function. Neuromolecular Med. 2005;7(1-2):79-99. doi: 10.1385/NMM:7:1-2:079. PMID: 16052040.
- Biancheri P, Di Sabatino A, Corazza GR, MacDonald TT. Proteases and the gut barrier. Cell Tissue Res. 2013 Feb;351(2):269-80. doi: 10.1007/s00441-012-1390-z. Epub 2012 Mar 20. PMID: 22427120.
- Budzyński J, Kłopocka M. Brain-gut axis in the pathogenesis of Helicobacter pylori infection. World J Gastroenterol. 2014 May 14;20(18):5212-25. doi: 10.3748/wjg.v20.i18.5212. PMID: 24833851; PMCID: PMC4017036.
- Filpa V, Moro E, Protasoni M, Crema F, Frigo G, Giaroni C. Role of glutamatergic neurotransmission in the enteric nervous system and brain-gut axis in health and disease. Neuropharmacology. 2016 Dec;111:14-33. doi: 10.1016/j.neuropharm.2016.08.024. Epub 2016 Aug 22. PMID: 27561972.
- Blachier F, Boutry C, Bos C, Tomé D. Metabolism and functions of L-glutamate in the epithelial cells of the small and large intestines. Am J ClinNutr. 2009 Sep;90(3):814S-821S. doi: 10.3945/ajcn.2009.27462S. Epub 2009 Jul 1. PMID: 19571215.
- Pál B. Involvement of extrasynaptic glutamate in physiological and pathophysiological changes of neuronal excitability. Cell Mol Life Sci. 2018 Aug;75(16):2917-2949. doi: 10.1007/s00018-018-2837-5. Epub 2018 May 15. PMID: 29766217.
- Tsai LH. Function of GABAergic and glutamatergic neurons in the stomach. J Biomed Sci. 2005;12(2):255-66. doi: 10.1007/s11373-005-1357-0. PMID: 15917994
- Xu J, Kurup P, Zhang Y, Goebel-Goody SM, Wu PH, Hawasli AH, Baum ML, Bibb JA, Lombroso PJ. Extrasynaptic NMDA receptors couple preferentially to excitotoxicity via calpain-mediated cleavage of STEP. J Neurosci. 2009 Jul 22;29(29):9330-43. doi: 10.1523/JNEUROSCI.2212-09.2009. PMID: 19625523; PMCID: PMC2737362.
- . De Vadder F, Grasset E, Mannerås Holm L, Karsenty G, Macpherson AJ, Olofsson LE, Bäckhed F. Gut microbiota regulates maturation of the adult enteric nervous system via enteric serotonin networks. Proc Natl AcadSci U S A. 2018 Jun 19;115(25):6458-6463. doi: 10.1073/pnas.1720017115. Epub 2018 Jun 4. PMID: 29866843; PMCID: PMC6016808.
- Koh A, De Vadder F, Kovatcheva-Datchary P, Bäckhed F. From Dietary Fiber to Host Physiology: Short-Chain Fatty Acids as Key Bacterial Metabolites. Cell. 2016 Jun 2;165(6):1332-1345. doi: 10.1016/j.cell.2016.05.041. PMID: 27259147.
- Fellows R, Denizot J, Stellato C, Cuomo A, Jain P, Stoyanova E, Balázsi S, Hajnády Z, Liebert A, Kazakevych J, Blackburn H, Corrêa RO, Fachi JL, Sato FT, Ribeiro WR, Ferreira CM, Perée H, Spagnuolo M, Mattiuz R, Matolcsi C, Guedes J, Clark J, Veldhoen M, Bonaldi T, Vinolo MAR, Varga-Weisz P. Microbiota derived short chain fatty acids promote histone crotonylation in the colon through histone deacetylases. Nat Commun. 2018 Jan 9;9(1):105. doi: 10.1038/s41467-017-02651-5. PMID: 29317660; PMCID: PMC5760624.
- Shi H, Kokoeva MV, Inouye K, Tzameli I, Yin H, Flier JS. TLR4 links innate immunity and fatty acid-induced insulin resistance. J Clin Invest. 2006 Nov;116(11):3015-25. doi: 10.1172/JCI28898. Epub 2006 Oct 19. PMID: 17053832; PMCID: PMC1616196.
- Anitha M, Vijay-Kumar M, Sitaraman SV, Gewirtz AT, Srinivasan S. Gut microbial products regulate murine gastrointestinal motility via Toll-like receptor 4 signaling. Gastroenterology. 2012 Oct;143(4):1006-16.e4. doi: 10.1053/j.gastro.2012.06.034. Epub 2012 Jun 23. PMID: 22732731; PMCID: PMC3458182
- Vargas-Caraveo A, Sayd A, Maus SR, Caso JR, Madrigal JLM, García-Bueno B, Leza JC. Lipopolysaccharide enters the rat brain by a lipoprotein-mediated transport mechanism in physiological conditions. Sci Rep. 2017 Oct 13;7(1):13113. doi: 10.1038/s41598-017-13302-6. PMID: 29030613; PMCID: PMC5640642
- Chen Z, Jalabi W, Shpargel KB, Farabaugh KT, Dutta R, Yin X, Kidd GJ, Bergmann CC, Stohlman SA, Trapp BD. Lipopolysaccharide-induced microglial activation and neuroprotection against experimental brain injury is independent of hematogenous TLR4. J Neurosci. 2012 Aug 22;32(34):11706-15. doi: 10.1523/JNEUROSCI.0730-12.2012. PMID: 22915113; PMCID: PMC4461442
- Kaushik DK, Gupta M, Das S, Basu A. Krüppel-like factor 4, a novel transcription factor regulates microglial activation and subsequent neuroinflammation. J Neuroinflammation. 2010 Oct 15;7:68. doi: 10.1186/1742-2094-7-68. PMID: 20946687; PMCID: PMC2965135.
- Louveau A, Herz J, Alme MN, Salvador AF, Dong MQ, Viar KE, Herod SG, Knopp J, Setliff JC, Lupi AL, Da Mesquita S, Frost EL, Gaultier A, Harris TH, Cao R, Hu S, Lukens JR, Smirnov I, Overall CC, Oliver G, Kipnis J. CNS lymphatic drainage and neuroinflammation are regulated by meningeal lymphatic vasculature. Nat Neurosci. 2018 Oct;21(10):1380-1391. doi: 10.1038/s41593-018-0227-9. Epub 2018 Sep 17. PMID: 30224810; PMCID:PMC6214619.
- Morimoto K, Nakajima K. Role of the Immune System in the Development of the Central Nervous System. Front Neurosci. 2019 Sep 3;13:916. doi: 10.3389/fnins.2019.00916. PMID: 31551681; PMCID: PMC6735264.
- Thion MS, Low D, Silvin A, Chen J, Grisel P, Schulte-Schrepping J, Blecher R, Ulas T, Squarzoni P, Hoeffel G, Coulpier F, Siopi E, David FS, Scholz C, Shihui F, Lum J, Amoyo AA, Larbi A, Poidinger M, Buttgereit A, Lledo PM, Greter M, Chan JKY, Amit I, Beyer M, Schultze JL, Schlitzer A, Pettersson S, Ginhoux F, Garel S. Microbiome Influences Prenatal and Adult Microglia in a Sex-Specific Manner. Cell. 2018 Jan 25;172(3):500-516.e16. doi: 10.1016/j.cell.2017.11.042. Epub 2017 Dec 21. PMID: 29275859; PMCID: PMC5786503
- denBesten G, van Eunen K, Groen AK, Venema K, Reijngoud DJ, Bakker BM. The role of short-chain fatty acids in the interplay between diet, gut microbiota, and host energy metabolism. J Lipid Res. 2013 Sep;54(9):2325-40. doi: 10.1194/jlr.R036012. Epub 2013 Jul 2. PMID: 23821742; PMCID: PMC3735932.
- Radak Z, Zhao Z, Koltai E, Ohno H, Atalay M. Oxygen consumption and usage during physical exercise: the balance between oxidative stress and ROS-dependent adaptive signaling. Antioxid Redox Signal. 2013 Apr 1;18(10):1208-46. doi: 10.1089/ars.2011.4498. Epub 2012 Nov 16. PMID: 22978553; PMCID: PMC3579386.
- Igarashi and Guarente, 2016;Igarashi M, Guarente L. mTORC1 and SIRT1 Cooperate to Foster Expansion of Gut Adult Stem Cells during Calorie Restriction. Cell. 2016 Jul 14;166(2):436-450. doi: 10.1016/j.cell.2016.05.044. Epub 2016 Jun 23. PMID: 27345368.
- Wang et al., 2016;Wang G, Yao J, Li Z, Zu G, Feng D, Shan W, Li Y, Hu Y, Zhao Y, Tian X. miR-34a-5p Inhibition Alleviates Intestinal Ischemia/Reperfusion-Induced Reactive Oxygen Species Accumulation and Apoptosis via Activation of SIRT1 Signaling. Antioxid Redox Signal. 2016 Jun 10;24(17):961-73
- Velloso LA, Folli F, Saad MJ. TLR4 at the Crossroads of Nutrients, Gut Microbiota, and Metabolic Inflammation. Endocr Rev. 2015 Jun;36(3):245-71. doi: 10.1210/er.2014-1100.
- Hsiao EY, McBride SW, Hsien S, Sharon G, Hyde ER, McCue T, Codelli JA, Chow J, Reisman SE, Petrosino JF, Patterson PH, Mazmanian SK. Microbiota modulate behavioral and physiological abnormalities associated with neurodevelopmental disorders. Cell. 2013 Dec 19;155(7):1451-63.
- Tillisch K, Labus J, Kilpatrick L, Jiang Z, Stains J, Ebrat B, Guyonnet D, Legrain-Raspaud S, Trotin B, Naliboff B, Mayer EA. Consumption of fermented milk product with probiotic modulates brain activity. Gastroenterology. 2013 Jun;144(7):1394-401, 1401.e1-4.
- Gareau MG, Wine E, Rodrigues DM, Cho JH, Whary MT, Philpott DJ, Macqueen G, Sherman PM. Bacterial infection causes stress-induced memory dysfunction in mice. Gut. 2011 Mar;60(3):307-17. doi: 10.1136/gut.2009.202515. Epub 2010 Oct 21. PMID: 20966022.
- Buffington SA, Di Prisco GV, Auchtung TA, Ajami NJ, Petrosino JF, Costa-Mattioli M. Microbial Reconstitution Reverses Maternal Diet-Induced Social and Synaptic Deficits in Offspring. Cell. 2016 Jun 16;165(7):1762-1775. doi: 10.1016/j.cell.2016.06.001.
- Kato-Kataoka A, Nishida K, Takada M, Kawai M, Kikuchi-Hayakawa H, Suda K, Ishikawa H, Gondo Y, Shimizu K, Matsuki T, Kushiro A, Hoshi R, Watanabe O, Igarashi T, Miyazaki K, Kuwano Y, Rokutan K. Fermented Milk Containing Lactobacillus casei Strain Shirota Preserves the Diversity of the Gut Microbiota and Relieves Abdominal Dysfunction in Healthy Medical Students Exposed to Academic Stress. Appl Environ Microbiol. 2016 May 31;82(12):3649-58. doi: 10.1128/AEM.04134-15.
- Messaoudi M, Violle N, Bisson JF, Desor D, Javelot H, Rougeot C. Beneficial psychological effects of a probiotic formulation (Lactobacillus helveticus R0052 and Bifidobacterium longum R0175) in healthy human volunteers. Gut Microbes. 2011 Jul-Aug;2(4):256-61. doi: 10.4161/gmic.2.4.16108. E
- Mittal M, Siddiqui MR, Tran K, Reddy SP, Malik AB. Reactive oxygen species in inflammation and tissue injury. Antioxid Redox Signal. 2014 Mar 1;20(7):1126-67. doi: 10.1089/ars.2012.5149.
- Munoz-Bellido JL, Munoz-Criado S, Garcìa-Rodrìguez JA. Antimicrobial activity of psychotropic drugs: selective serotonin reuptake inhibitors. Int J Antimicrob Agents. 2000 Apr;14(3):177-80.
- Flowers SA, Evans SJ, Ward KM, McInnis MG, Ellingrod VL. Interaction Between Atypical Antipsychotics and the Gut Microbiome in a Bipolar Disease Cohort. Pharmacotherapy. 2017 Mar;37(3):261-267.
- Wang Y, Wang Z, Wang Y, Li F, Jia J, Song X, Qin S, Wang R, Jin F, Kitazato K and Wang Y (2018) The Gut-Microglia Connection: Implications for Central Nervous System Diseases. Front. Immunol. 9:2325.
- Geng ZH, Zhu Y, Li QL, Zhao C, Zhou PH. Enteric Nervous System: The Bridge Between the Gut Microbiota and Neurological Disorders. Front Aging Neurosci.