The Effects of Stem Cell Therapy in the Treatment of Childhood Acute Lymphoblastic Leukemia
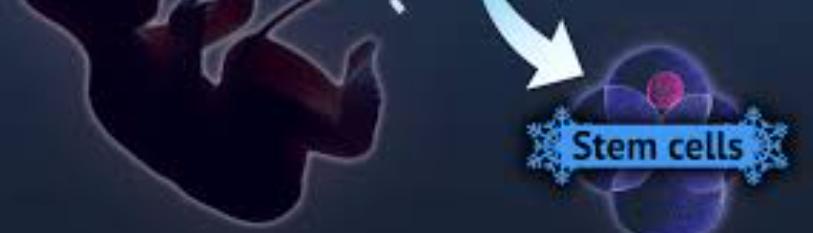
Emma Lawrence and Vincent S Gallicchio*
Department of Biological Sciences, College of Science, Clemson University, Clemson, USA
Corresponding author: Vincent S Gallicchio, Department of Biological Sciences, College of Science, Clemson University, Clemson, USA
Citation: Lawrence E, Gallicchio VS. (2020) The Effects of Stem Cells on Recovery after Stroke. J Stem Cell Res. 1(2):1-13.
Received: July 13, 2020 | Published: August 19, 2020
Copyright© 2020 genesis pub by Lawrence E, et al. CC BY-NC-ND 4.0 DEED. This is an open-access article distributed under the terms of the Creative Commons Attribution-Non Commercial-No Derivatives 4.0 International License., This allows others distribute, remix, tweak, and build upon the work, even commercially, as long as they credit the authors for the original creation.
DOI: https://doi.org/10.52793/JSCR.2020.1(2)-08
Abstract
Acute Lymphoblastic Leukemia (ALL) is a cancerous transformation in the blood and bone marrow beginning with progenitor cells. With developing research into treatment options, there is now a 90% survival rate. However, high relapse rates have become the next challenge to overcome. Relapse rates increase with a variety of markers including age of diagnosis, white blood cell counts, and response to chemotherapy. Chemotherapy and radiation have been the standard of care in the past, but are typically accompanied by secondary malignancies later in life. Researchers believe that stem cell therapies may offer alternative options to high-risk patients.
Keywords
Stem cells; Therapy; Childhood ALL
Abbreviations
ALL: Acute Lymphoblastic Leukemia; RBCs: Red blood cells; CBC: Complete Blood Count; HR: High Risk; VHR: Very High Risk; WBC: White Blood Cell; EFS: Event Free Survival; CAR: Chimeric Antigen Receptor; MUD: Matched Unrelated Donor; MRD: Matched Related Donor; GvHD: Graft vs. Host Disease; TBI: Total Body Irradiation; ASC: Adult Stem Cells; ESC: Embryonic Stem Cells; iPSC: Induced Pluripotent Stem Cells; HSCT: Hematopoietic Stem Cell Transplant; MLL: Mixed-lineage-Leukemia; NK: Natural Killer; NRM: Non-remission mortality; VHRR: Very High Risk of Relapse
Introduction
Acute Lymphoblastic Leukemia (ALL) is a rare type of leukemia in adults, but the most common type found in children, peaking between the ages of two and five [1]. It is a rapidly progressing cancer that occurs when errors in DNA cause bone marrow cells to continue to grow and divide when they would normally stop. These abnormal cells are unable to mature into proper white blood cells and eventually crowd out healthy cells. Risk factors of developing ALL include previous cancer treatments, radiation exposure, genetic disorders, and a family history [2].
In its maturation, a blood stem cell will become a myeloid stem cell or a lymphoid stem cell. Myeloid stem cells can then develop into a red blood cell (to carry oxygen), white blood cell (to fight infection), or platelet (to form blood clots). A lymphoid stem cell may become a B lymphocyte (to make antibodies), T lymphocyte (to help make B lymphocytes), or a Natural Killer cell (to attack cancer cells and viruses). ALL is confirmed when too many stem cells becoming lymphoblasts, B lymphocytes, and T lymphocytes. This change is unfavorable because these cells cannot fight infection well. Genetic risk factors include Down syndrome, Neurofibromatosis type 1, Bloom syndrome, Fanconi anemia, Li-Fraumeni syndrome, Ataxia-telangiectasia, and errors in the mismatch repair system [3] (Figure 1).
Figure 1: Shows the normal development of a stem cell into RBC’s, platelets, granulocytes, monocytes, and lymphocytes. In ALL, lymphoid stem cells divide abnormally leading to cancer [4].
Signs of ALL include fever, easy bruising, petechiae, bone and joint pain, lumps in the neck, underarm, stomach and groin, weakness, and loss of appetite. A diagnosis can be confirmed by a complete blood count (CBC), blood chemistry study, bone marrow biopsy, or cytogenic analysis (analysis of chromosome rearrangements). Prognosis factors include age at diagnosis, whether leukemia cells came from B or T lymphocytes, chromosome rearrangements, and how quickly leukemia cell counts drop in the first month. There are three risk groups for childhood ALL. The standard risk group includes children age 1-10 who have a WBC< 50,000 uL. The high-risk (HR) group includes children age 10 and older or a WBC> 50,000 u. The very high-risk (VHR) group includes children less than 1 year old, those with certain gene abnormalities, children who have a slow response to treatment, and those who continue to show signs of leukemia after 4 weeks of chemotherapy [3].
Treatment options vary based on diagnosis factors, but include chemotherapy, radiation therapy, stem cell therapy, and target therapy [3]. Chemotherapy can be given systemically or regionally, depending on the categorized risk group. High-risk children receive higher doses and more aggressive treatments than standard risk patients. Radiation therapy may be administered externally or internally. External radiation is used to treat ALL that has spread, or may spread, to various areas of the body, most notably the brain and spinal cord. It may also be used in preparation for a stem cell transplant. Stem cell transplants may be used to replace healthy blood-forming cells after treatments of chemotherapy and total-body irradiation. Stem cells are typically used only for cases in which there is a high risk of relapse [5-7]. Additionally, many target therapies show promise in the treatment of childhood ALL including tyrosine kinase inhibitors, monoclonal antibody therapy, and proteasome inhibitor therapy [3].
Treatment options vary based on diagnosis factors, but include chemotherapy, radiation therapy, stem cell therapy, and target therapy [3]. Chemotherapy can be given systemically or regionally, depending on the categorized risk group. High-risk children receive higher doses and more aggressive treatments than standard risk patients. Radiation therapy may be administered externally or internally. External radiation is used to treat ALL that has spread, or may spread, to various areas of the body, most notably the brain and spinal cord. It may also be used in preparation for a stem cell transplant. Stem cell transplants may be used to replace healthy blood-forming cells after treatments of chemotherapy and total-body irradiation. Stem cells are typically used only for cases in which there is a high risk of relapse [5-7]. Additionally, many target therapies show promise in the treatment of childhood ALL including tyrosine kinase inhibitors, monoclonal antibody therapy, and proteasome inhibitor therapy [3].
Variable |
Favorable Variable |
Adverse Factor |
Use in Risk Stratification |
Demographic and clinical features |
|||
Age |
1 to <10 yr |
<1 yr or ≥10 yr |
This feature is a part of NCI risk group definition |
Sex |
Female |
Male |
No |
Race or ethnic group |
White, Asian |
Black, Native American, Hispanic |
No |
Initial white-cell count |
Lower (<50,000/mm³) |
Higher (≥50,000/mm³) |
Part of NCI risk group definition |
Biological or genetic features of Leukemia cell |
|||
Immunophenotype |
B-cell lineage |
T-cell lineage |
Often used to select therapy backbone |
Cytogenetic features |
ETV6-RUNX1, hyperdiploidy, favorable chromosome trisomies |
BCR-ABL1, MLL rearrangements, hypodiploidy |
Often used to select treatment intensity, assign the patient HSCT, or both; some features (e.g., BCR-ABL1) can be used to select targeted therapy |
Genomic features |
ERG deletions |
IKZF1 deletions or mutations; Philadelphia chromosome- like ALL with kinase gene alterations |
Some research groups use IKZF1 deletions to assign patients to more intensive therapy; kinase gene mutations may be used to assign patients to targeted therapy, but this is not yet part of routine care |
Early response to treatment |
|||
Response to 1 week of glucocorticoid therapy |
Good response to prendisone (<1000 blasts/mm³) |
Poor response to prendisone (≥1000 blasts/mm³) |
Easy to measure and used by many groups; may be supplanted by MRD |
Marrow blasts after 1-2 weeks of multiagent therapy |
M1 marrow (<5% blasts) by day 8 or 15 |
No M1 marrow (≥5% blasts) by day 8 or 15 |
Easy to measure and used previously by many groups; now being supplanted by MRD |
MRD quantitation during or at end of induction |
Reaching low (<0.01%) or undetectable MRD by specific time points |
Persistence of MRD ≥0.01% at specific time points; the higher it is, the worse the prognosis |
Most important single prognostic factor for contemporary therapy; critical for modern risk stratification |
MRD at 3-4 months |
Low (<0.01%); preferably undetectable |
Persistence of MRD ≥0.01% |
May help select patients for HSCT or new therapies in first remission |
*HSCT denotes hematopoietic stem-cell transplant, MRD minimal residual disease, and NCI National Cancer Institute |
Table 1: Shows important prognosis factors in Childhood Acute Lymphoblastic Leukemia. These factors include age, sex, ethnicity, initial WBC, genomic factors, and responses to treatment. Determining these factors for a patient leads to a risk level analysis [11].
Two subtypes of childhood ALL are B-ALL and T-ALL. B-ALL makes up 85% of pediatric cases, while T-ALL accounts for 10-15% of pediatric cases and results in poor early treatment responses and high rates of relapse [12,13]. Current treatment of T-ALL involves the use of chemotherapy drugs cyclophosphamide, mercaptopurine, and cytarabine, which have shown promising outcomes [13]. However, chemotherapy can lead to drug toxicity and side-effects including glucocorticoid-related osteonecrosis, Asparaginase-related pancreatitis, and vincristine-related peripheral neuropathy. Relapsed ALL is associated with a greater resistance to chemotherapy [14]. Anthracyclines are a type of antibiotic derived from Streptomyces bacteria that treat cancer by damaging the DNA in cancer cells. They have been shown to be effective against bone marrow relapse, but result in no significant increase in event free survival (EFS) for childhood ALL. Anthracycline can also result in cardiotoxicity and heart failure, which has led to treatment protocols of its use in selected high-risk cases [14-16]. Novel approaches to ALL treatment have led to CAR-T therapy, which involves the infusion of T-cells that have been genetically engineered to express the chimeric antigen receptor (CAR) in cells [17]. These T-cells tailor the body’s immune response against specific pathogens. CAR-T therapy could be an option to replace failed treatment for patients with relapsed or refractory B- ALL [17,18]. Melphalan and cyclophosphamide with etoposide have also shown high rates of EFS when paired with hematopoietic stem cell transplants (HSCT) from an alternative donor [19] (Figure 2).
Figure 2: Shows the distribution and rates of various genetic alterations leading to childhood ALL. Additionally, it compares the rates between the two subgroups of ALL: B-Cell lineage and T-Cell lineage [20].
HSCT has offered a promising treatment option to induce antileukemic control in children with ALL who don’t respond favorably to current chemotherapy approaches [21]. Two types of donor groups include matched unrelated donor (MUD) and matched related donor (MRD). Both MUD and MRD are classified as allogenic, meaning that they are derived from a matched, partially mismatched, or an unrelated donor. Reduced intensity allogenic stem cell treatment uses a lower dose of chemotherapy so the immune system isn’t completely inactivated, which can lead to better outcomes for some older and sicker patients [22]. Allogenic transplants however, do lead to a heightened risk of graft-vs-host disease (GvHD), where the donors’ immune cells attack the recipients normal tissue. GvHD presents with skin rashes, jaundice, severe diarrhea, fatigue, and muscle aches [22,23]. Immunosuppressant’s like cyclosporine are administered following transplant to prevent GvHD, but can leave the body vulnerable to infection24,25. Steroids in combination with calcineurin inhibitor are currently used to treat GvHD. Conversely, autologous transplants are when a patient receives their own stem cells (previously frozen), but is not a typical option due to its association with a high-relapse rate and the risk that the stem-cells are still contaminated with leukemia cells. In most cases, a matching related donor can’t be found, so alternative approaches to allo-HSCT are used, including umbilical cord blood and unrelated/haploidentical allo-HSCT [24,26] (Figure 3).
Figure 3: Compares and contrasts the procedures for an autologous vs. allogenic stem cell transplant. These differences lead to different outcomes in patients (ie., risk of GvHD) [27].
Total body irradiation has been associated with the late onset of other complications, including cardiac, pulmonary, and renal complications; cataract; and a heightened risk of developing secondary malignancies. These secondary malignancies include organ dysfunction, growth retardation, as well as hormonal imbalances [28]. Cognitive impairments and developmental abnormalities have caused concerns for TBI in pediatric patients. Treatment without TBI has shown comparable results by using busulfan and cyclophosphamide in addition to HSCT, eliminating the adverse effects associated with TBI. This regimen has provided promising results in pediatric and infant ALL [19].
Stem cells are in a broad classification of which all other cells derive from. Once they divide into daughter cells, they can become new stem cells or differentiate into a more specific function (blood cells, brain cells, heart muscle, etc.). Different types of stem cells include adult stem cells (ASCs), embryonic stem cells (ESCs), cord blood/amniotic fluid stem cells, and induced pluripotent stem cells (iPSC’s) [29].
Embryonic stem cells are derived from 3-5 day old embryos, and are pluripotent, while making up about 0.01-0.2% of total mononuclear bone marrow cells in humans. They are collected by the process of in-vitro fertilization, which occurs in a lab. ESC’s are grown in test tubes or Petri dishes, typically to later be used during testing of new drugs for safety and effectiveness. These stem cells are defined by their origin in the inner cell mass of the blastocyst [29].
Adult stem cells are derived from a multitude of different tissues, and are more limited in their ability to differentiate into specific tissues. Like all stem cells, they have the ability to make identical copies of themselves in the process of long-term self-renewal. The undifferentiated cell is known as a progenitor cell, or precursor. To be classified as an ASC, the cell must be efficient at self-renewal for an organisms’ entire lifespan. It also must be clonogenic, meaning it can give rise to a lineage of genetically identical cells [29].
Recent developments have been able to genetically reprogram adult stem cells to act as if it were an embryonic stem cell. These cells are called iPSC’s, and can differentiate into all types of specialized cells to produce new cells, organs, or tissues. iPSC’s are derived from a patient’s skin or blood before being reprogrammed back into ESC’s. These stem cells have offered an option for treatment without the ethical issues surrounding ESC’s because the stem cells come from the patient. This option is also safer because it limits the possibility of rejection [30]. There is a preference for the use of embryonic stem cells over adult stem cells due to their versatility, but due to iPSCs, adult stem cells have shown new potential. In HSCT in the treatment of ALL, adult stem cells or umbilical cord blood are used to replace cells damaged by chemotherapy or disease [29].
A stem cell transplant is suggested in rare cases, including those in which ALL shows a likelihood of coming back (high risk), has come back (relapse ALL), or is no longer responding to standard treatment. In preparation for a HSCT, chemotherapy is first administered to kill leukemia cells, killing health cells in bone marrow in the process, making room for stem cells following transplant. Chemotherapy works to dampen the immune system, preventing the host from rejecting the donor stem cells following transplantation6. Long-term side effects of HSCT include the growth of another cancer, lung problems, lack of a menstrual period (damage to ovaries), and vision problems (damage to the lens of the eye) [23].
HSCT has been found to be a valuable course of action for high risk patients and a subgroup of infant mixed-lineage-leukemia (MLL+) ALL that carry poor prognosis factors [28,31]. These prognosis factors included infants being below the age of 6 months, prendiso(lo)ne response (poor predniso(lo)ne response [PPR]: ≥ 1.0 g/L blasts at day 8), and WBC counts in peripheral blood at diagnosis (≥ 300 g/L vs < 300 g/L). This study found that 80% of infant cases harbor rearrangements in the MLL gene. Because of the high risks in infant ALL, HSCT survivors can face various other chronic conditions [28].
The information together regarding the MLL arrangement and ALL’s peak between ages 2 and 5 supports the theory that additional exogenous exposures play a part in the development of ALL. Endogenous exposures influencing ALL development include inflammation and oxidative stress, while exogenous exposures include an abnormal response to infection. The influenza virus is a promising candidate as an exogenous exposure. Other chromosomal abnormalities also increase the risk of ALL, including Trisomy 21 (down syndrome), hyperploidy, and translocations. All of these rearrangements are related to a dismal prognosis. Additionally, gene mutations of IKZF1, ARID5B, CEBPE, and CDKN2A have been associated with the occurrence of ALL due to their role as key regulators of blood development, proliferation, and differentiation. Racial and ethnic differences due to socioeconomic factors as well as genetic differences cause a disparity in the occurrence of ALL. Ancestry related genetic variations have led to a heightened occurrence of ALL in individuals with Hispanic ethnicity, followed by those of Asian Pacific and European Descent, and finally those of African ethnicity [31] (Table 2).
Representative Genetic Alterations or Subtypes |
||||
Favorable outcomes- standard therapy |
-Hyperdiploidy -ETV6-RUNX1 |
Diagnosis |
Therapy |
Remission |
Improved outcomes- intensified therapy |
-TCF3-PBX1 -BCR-ABL1 -T-ALL |
Diagnosis |
Therapy |
Remission |
Chemoresistance and/or relapse- due to inherent genetic alterations |
-Hypodiploidy -KMT2A rearrangement -iAMP21 -Ph-like |
Diagnosis |
Therapy |
Chemorefractory ALL/ relapse |
Chemoresistance leading to relapse- due to acquired genetic alterations |
-CREBBP mutations -NT5C2 mutations -ABL1 "gatekeeper" mutation |
Diagnosis |
Therapy |
Chemorefractory ALL/ relapse |
Table 2: Genetic alterations lead to variable outcomes based on responses to chemotherapy. The first two boxes represent chemosensitive ALL, while the second two boxes represent chemoresistant ALL. Chemoresistant ALL may develop from drug-resistant subclones, leading to the development of acquired mutations and later relapse [32].
In animal trials, mouse models have been used due to mice having at disease manifestation of ALL similar to that of humans. Xenograph models have been used on mice to analyze original patient samples for disease characterization and evaluation for potential therapeutics. Limitations to the xenograph model approach include the fact that the immunosuppressants represent a separate environment to that of human. Normal immune cells are usually infused in mice for pre-clinical immunotherapy studies. Genetic murine models are another approach to ALL in animal studies. Many of these models manifest the symptoms of human ALL including leukemia cell accumulation in the thymus, lymph nodes, bone marrow, spleen, and infiltration in the liver and brain. In ras transgenic mice, ALL developed spontaneously, and half of the mice acquired a NOTCH1 gain of function mutation which led to accelerated T-ALL onset and decreased survival rate. NOTCH proteins are transmembrane receptors involved in cell development and in the transcriptional activation of target gees. This information suggests that NOTCH1 is a driving force in T-cell transformation in ALL. Animal models have shown to be an excellent way to study GvHD in transplants. Canine models have been essential in the testing of immunosuppressant therapies following transplantation. Methotraxate is an example, as it was first tested in dogs and was found to be effective in reducing the occurrence of GvHD33.
In human trials, CD16+ Natural Killer cells (NK) from cord blood stem cells have been used in cancer therapy by using an anti-CD47 antibody. This antibody triggers an antitumor response [34]. With ALL showing a higher level of CD47, the cord blood stem cells eradicated the ALL cells by using this antibody. Additional human trials of HSCT in patients found that transplants before a third or subsequent complete remission had better survival rates. Patients with transplants after a second complete remission had a 2.3 times greater risk of death [35].
HSCT from an allogenic donor is now the standard of care for the high risk subcategory, a qualification applying to only 10% of ALL patients [22]. The option of using HLA-matched unrelated donors (MUDs) has increased the availability to use stem cell transplants. In the Berlin-Frankfurt-Muenster study group, patients received HSCT from either a MUD or matched sibling donor (MSD). They found that engraftment was faster in the MSD-HSCT transplant group, but chronic GvHD also occurred more in this group and was more extensive in patients older than 12. This increase in occurrence could have been from the MUD group receiving more intensive immunosuppressant’s. Graft failure was rare, but occurred more often in those receiving transplants from a MUD. Superior outcomes were attributed to the MSD group due to rapid immune reconstruction and fewer infections, but MUD had highly successful outcomes as well. MUD-HSCT transplants could be applied to patients without a suitable related donor [24].
HSCT results have improved through a decrease in nonremission mortality (NRM). Relapse is not uncommon, with rates or relapse reaching 20% following the first complete remission. These high relapse patients are eligible for MSD of MUD HSCT, along with those who are VHRR (very high relapse risk) [7]. These patients receive hyperfractioned TBI followed by immunosuppressant’s, which can be ceased if there is no occurrence of GvHD after 100 days [25]. HSCT from both related and unrelated donors has been associated with non-remission mortality and relapse. For this reason, HSCT is limited to patients of high-risk. Relapse has been the most difficult issue to address in childhood ALL due to it commonly being associated with resistance to chemotherapy. Variations of immunomodulation and tailored chemotherapy are plausible ways to decrease the risk of relapse [7].
Conclusion
Acute Lymphoblastic Leukemia is a cancer of the blood and bone marrow caused by the inability of progenitor cells to replicate properly. A high-risk factor indicative of ALL is the occurrence of chromosomal translocations and replications [36]. While ALL survival rates have reached 90%, relapse rates remain high [37]. Survival depends on a number of factors including the age of diagnosis, white blood cell count, and response to chemotherapy [36].
In attempts to treat patients following relapse, researchers have looked to stem cell use. Both MRD and MUD stem cell transplants have shown to be potential options. HSCT’s correlate with a heightened risk of secondary malignancies, leading transplants to be a valuable option only to a specific subgroup of ALL who are at a heightened risk of relapse [28]. While studies have supported the use of HSC’s in the treatment of ALL, there is more research to be done in the hopes of reducing both secondary malignancies and relapse.
References
- Inaba H, Greaves M, Mullighan CG. (2013) Acute lymphoblastic leukaemia. The Lancet. 381(9881):1943-1955
- Acute lymphocytic leukemia - Symptoms and causes - Mayo Clinic (2020) . Available: https://www.mayoclinic.org/diseases-conditions/acute-lymphocytic-leukemia/symptoms-causes/syc-20369077
- PDQ Pediatric Treatment Editorial Board. Childhood Acute Lymphoblastic Leukemia Treatment (PDQ®): Patient Version.; 2002. (2020) Available: http://www.ncbi.nlm.nih.gov/pubmed/26389385
- What is acute lymphocytic leukemia? - Canadian Cancer Society. (2020) Available: https://www.cancer.ca/en/cancer-information/cancer-type/leukemia-acute-lymphocytic-all/acute-lymphocytic-leukemia/?region=on
- Mattson K, Honkaniemi E, Ramme E, Brabany G, Sander BM, et al. (2019) Strong expression of p53 protein in bone marrow samples after hematopoietic stem cell transplantation indicates risk of relapse in pediatric acute lymphoblastic leukemia patients. Pediatr Transplant. 23(4):e13408.
- https://www.cancerresearchuk.org/about-cancer/acute-lymphoblastic-leukaemia-all/treatment/bone-marrow-stem-cell-transplant/what-is-stem-cell-or-bone-marrow-transplant
- Dalle JH, Balduzzi A, Bader P, et al. (2018) Allogeneic Stem Cell Transplantation from HLA-Mismatched Donors for Pediatric Patients with Acute Lymphoblastic Leukemia Treated According to the 2003 BFM and 2007 International BFM Studies: Impact of Disease Risk on Outcomes. Biol Blood and Marrow Transplantation.24(9):1848-1855.
- Tasian SK, Loh ML, Hunger SP. (2015) Childhood acute lymphoblastic leukemia: Integrating genomics into therapy. Cancer. 121(20):3577-3590.
- Pui CH, Yang JJ, Bhakta N, Rodriguez-Galindo C. (2018) Global efforts toward the cure of childhood acute lymphoblastic leukaemia. The Lancet Child and Adolescent Health. 2(6):440-454.
- Hunger SP, Mullighan CG. (2015) Acute lymphoblastic leukemia in children. Longo DL, ed. New England Journal of Medicine. 373(16):1541-1552.
- Tasian SK, Loh ML, Hunger SP. (2015) Childhood acute lymphoblastic leukemia: Integrating genomics into therapy. Cancer. 121(20):3577-3590.
- Cyclophosphamide- National Cancer Institute. Accessed July 1, 2020. Available: https://www.cancer.gov/about-cancer/treatment/drugs/cyclophosphamide
- Aberuyi N, Rahgozar S, Ghodousi ES, Ghaedi K. (2020) Drug Resistance Biomarkers and Their Clinical Applications in Childhood Acute Lymphoblastic Leukemia. Front Oncol. 9:1496.
- Definition of anthracycline - NCI Dictionary of Cancer Terms - National Cancer Institute. (2020) Available: https://www.cancer.gov/publications/dictionaries/cancer-terms/def/anthracycline
- Eden TOB, Pieters R, Richards S. (2009) Beneficial and harmful effects of anthracyclines in the treatment of childhood acute lymphoblastic leukaemia: A systematic review and meta-analysis. Br J Haemato. 145(3):376-388.
- Zhuo Y, Wang J, Lu A. Wu L, Dong L. et al. (2016) Chimeric antigen receptors T cells in treatment of a relapsed pediatric acute lymphoblastic leukemia, relapse after allogenetic hematopoietic stem cell transplantation: case report and review of literature review]. Zhonghua Xue Ye Xue Za Zhi. 37(2):115-18.
- D’Aloia MM, Zizzari IG, Sacchetti B, Pierelli L, Alimandi M. (2018) CAR-T cells: The long and winding road to solid tumors review-article. Cell Death and Disease. 9(3):282.
- Kato M, Ishida H, Koh K, Inagaki J, Kato K, et al. (2015) Comparison of chemotherapeutic agents as a myeloablative conditioning with total body irradiation for pediatric acute lymphoblastic leukemia: A study from the pediatric ALL working group of the Japan Society for Hematopoietic Cell Transplantation. Pediatr Blood Cancer. 62(10):1844-50.
- http://www.cancer.gov
- https://reference.medscape.com/medline/abstract/25255163
- https://www.lls.org/leukemia/acute-lymphoblastic-leukemia/treatment/stem-cell-transplantation
- https://www.urmc.rochester.edu/encyclopedia/content.aspx?contenttypeid=34&contentid=BALLT12
- Peters C, Schrappe M, von Stackelberg A, et al. Stem-Cell Transplantation in Children With Acute Lymphoblastic Leukemia: A Prospective International Multicenter Trial Comparing Sibling Donors With Matched Unrelated Donors—The ALL-SCT-BFM-2003 Trial. Journal of Clinical Oncology. 2015;33(11):1265-1274. doi:10.1200/JCO.2014.58.9747
- https://www.medicinenet.com/graft_versus_host_disease_gvhd/article.htm
- https://www.mayoclinic.org/tests-procedures/bone-marrow-transplant/in-depth/stem-cells/art-20048117
- Allogeneic Hematopoietic Cell Transplantation For Multiple Myeloma.
- Hamidieh AA, Babaki AES, Rostami T, Kasaeian A, Koochakzadeh L, et al. (2019) A Single-Center Experience With Hematopoietic Stem Cell Transplantation for Pediatric Acute Lymphoblastic Leukemia: A Modest Pitch for Non-Total Body Irradiation Conditioning Regimens.Experim Clin Transp. 17(2):243-250.
- Stem cells: What they are and what they do - Mayo Clinic.
- The Promise of Induced Pluripotent Stem Cells (iPSCs) | stemcells.nih.gov.
- Inaba H, Greaves M, Mullighan CG. (2013) Acute lymphoblastic leukaemia. The Lancet. 381(9881):1943-1955.
- Tasian SK, Loh ML, Hunger SP. (2015) Childhood acute lymphoblastic leukemia: Integrating genomics into therapy. Cancer. 121(20):3577-3590.
- You Q, Su H, Wang J, Jiang J, Qing G, Liu H. (2018) Animal models of T-cell acute lymphoblastic leukemia. Journal of Bio-X Research. 2018;1(1):32-40.
- Valipour B, Abedelahi A, Naderali E, Velaei K, Movassaghpour A, et al. (2020) Cord blood stem cell derived CD16+ NK cells eradicated acute lymphoblastic leukemia cells using with anti-CD47 antibody. Life Sciences. 242:117223.
- Gabelli M, Zecca M, Messina C, Carraro E, Buldini B, et al. (2019) Hematopoietic stem cell transplantation for isolated extramedullary relapse of acute lymphoblastic leukemia in children.Bone Marrow Transplant. 54(2):275-283.
- Beneficial and harmful effects of anthracyclines in the treatment of childhood acute lymphoblastic leukaemia: a systematic review and meta-analysis. Br J Haematol. 145(3):376-388.
- Inaba H, Greaves M, Mullighan CG. (2013) Acute lymphoblastic leukaemia. The Lancet. 381(9881):1943-1955.
- Kato M, Ishida H, Koh K, Inagagki J, Kato K, et al. (2020) Comparison of chemotherapeutic agents as a myeloablative conditioning with total body irradiation for pediatric acute lymphoblastic leukemia: A study from the pediatric ALL working group of the Japan Society for Hematopoietic Cell Transplantation. Pediatr Blood Cancer. 62(10):1844-50.