Thanks Mom! The Evolution-Health Disparities Link Through Mitochondrial Genetic Disease
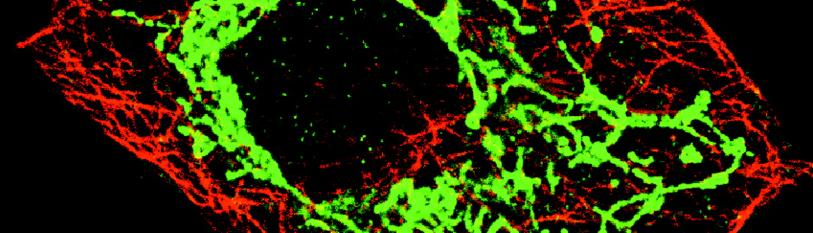
Fatimah Jackson1,2*, Elizabeth Mingo1,2, Jennifer Caldwell3, Naima Batson4,2, Meram Mohammed1,2 and Kaitlin Keaton1,2
1Department of Biology, Howard University, Washington DC
2Human Analytics Section, QuadGrid Data Lab, Bethesda, MD
3Pennington Biomedical Research Institute, Louisiana State University Baton Rouge, LA
4Charles Drew College of medicine, UCLA, Loss Angeles, CA
*Corresponding author: Fatimah Jackson, Department of Biology, Howard University and Human Analytics Section, QuadGrid Data Lab
Citation: Jackson F, Mingo E, Caldwell J, Batson N, Mohammed M, et al. (2023) Thanks Mom! The Evolution-Health Disparities Link Through Mitochondrial Genetic Disease. Adv Clin Med Res. 4(4):1-22.
Received: August 14, 2023 | Published: September 28, 2023
Copyright© 2023 genesis pub by Jackson F, et al. CC BY-NC-ND 4.0 DEED. This is an open-access article distributed under the terms of the Creative Commons Attribution-Non Commercial-No Derivatives 4.0 International License., This allows others distribute, remix, tweak, and build upon the work, even commercially, as long as they credit the authors for the original creation.
DOI: https://doi.org/10.52793/ACMR.2023.4(4)-66
Abstract
Anatomically modern humans evolved in Africa ~300,000 years ago. For this reason, the evolution of mtDNA genetic variants tracks the migratory histories of humanity worldwide. With these genetic variants have come corresponding diversity in mtDNA-associated genetic diseases. These tend to aggregate in ethnic and regional groups in conjunction with the concentration of specific mtDNA haplotypes in these same groups. Given the ubiquity of mtDNA in every human cell, the fact that mtDNA molecules encode genes for the mitochondrial respiratory chain, protein synthesis, and regulation, are of primarily maternal heritage, and do not undergo recombination, it is not surprising that mtDNA genetic diversity in combination with environmental factors influences health disparities.We provide a model for this interaction and a comprehensive overview of the range of genetic diseases impacted by specific mtDNA variants."95 cases were reviewed" covering 29 clinical conditions. 62% of all cases were in patients of European descent, 34% in individuals of East Asian descent, and only 1% were among people of recent African descent. mtDNA-associated diseases included diverse cancers, neurodegenerative diseases, and metabolic diseases. Many more adverse associations were reported than preventative. These findings are discussed within an evolutionary context.
Keywords
Human evolution; mtDNA cancer; Neurodegenerative; Metabolic diseases
Introduction
Genomic evolution in the homeland of humanity
Africa presents the most complex genetic picture of any continent, with a time depth for mitochondrial DNA (mtDNA) lineages >100,000 years [1]. Studies of human mtDNA genomes demonstrate that the root of the human phylogenetic tree is solidly in Africa [2]and comparison of the intra population sequence divergence in African and non-African populations confirms that African populations exhibit the largest extent of mtDNA variation. This observation supports the hypothesis that Africans represent the most ancient human group and that all modern humans have a common and recent African origin [3].
While humankind’s origins in Africa are thus well established in the scientific literature, the intra-continental migrations of these early humans around the African continent are less well documented. Most migration studies are one-dimensional and refer to the migration out of Africa of a subset of African humans approximately 60,000 years ago[4].Studies of mtDNA phylogeny within continental Africans suggest that the L0-L1’6 split occurred at about 140,000-160,000 years ago and may represent also an early sub-structuring of small, isolated, but increasingly mobile African communities [5]. As indigenous communities grew and dispersed geospatially within the African continent, regional variation accumulated over the following millennia, with L2 and L3 lineages arising in Central and East Africa ~100,000-75,000years ago. Their sub-Saharan dispersal prior to~60,000, largely overwhelmed the L0’1 distribution [5]. We still see evidence of this pattern of replacement in the early 21st century Ely-Jackson database of African and African American mtDNA variants [6].
It has been suggested that cyclic expansions and retractions of the equatorial forests in Africa between 40,000 years ago and the Last Glacial Aridity Maximum (21,000 years ago) caused in Africa a major expansion of existing deserts [7] along with a large drop in sea levels. These induced significant climatic shifts and reduced the genetic diversity of modern humans. Surviving regional-specific lineages emerged from the Sahelian refuge areas, including the Nile Valley [8], repopulating the region and contributing to the overall pattern of genetic similarity in West Africans. Subsequently, particular L1- L3 lineages expanded in range due to the substantial population growth made possible by moister and warmer conditions of the African humid period.
The African humid period extended over the Sahara as well as the entirety of eastern, southeastern and equatorial Africa [86] During this period, important technological advances to subsistence patterns emerged and were associated with the development of agriculture and implementation of iron smelting techniques that supported population growth and increased survival. During the African humid period, lakes, rivers, wetlands and vegetation including grass and trees covered the Sahara and Sahel [85, 10, 11] creating a Green Sahara with forests and woodlands expanding through the entire African continent. As recently as6,000 years ago the vast Sahara Desert was covered in grassland that received an abundance of rainfall. However, shifts in the world's weather patterns precipitated by fluctuations in the orientation of the orbit of the earth and changes in climate that abruptly transformed the well vegetated region into some of the most arid land on the planet. V. Černý and colleagues [12] suggested that the mtDNAL3 sub lades L3f2 and L3e5 are unique to the Chad Basin. The Bantu expansion of agriculturalists occurred towards the end of the periods, perhaps stimulated in part by the climatic changes. The diffusion of these farmers from West Central Africa toward South Africa around 3,000 years ago accelerated the replacement of much of the pre-existent mtDNA lineages among previously hunter-gatherer communities [13].
Critical to the retention of mtDNA diversity and the promotion of new variants has been the improved community carrying capacities associated with the shift from hunter-gatherer subsistence to agriculture. Researchers have observed changes in the pair wise sequence distributions, patterns of coalescence events, and numbers of variable positions relative to the mean sequence difference that suggest that Africans who remained hunter gatherers retained population sizes that stayed constant over time whereas those who embraced agriculture expanded in size [14]. We can also envision that those groups that remain as foragers would have been less likely to experience admixture [15] and in their relative isolation, retained more of the original genetic signatures. This hypothesis appears to be supported by recent studies of aDNA in Africa [15] that infer foragers have retained more of the original mtDNA sequences than agriculturalists and that within the last 5,000 years, population demographics in Africa have undergone significant rearrangements mediated by technological developments.
Natural selection and nonrandom mating practices alter mtDNA mutational loads
There are hundreds to thousands of copies of mitochondrial DNA (mtDNA) in each human cell in contrast to only two copies of nuclear DNA [16]. Molecular variants of mtDNA have three origins [17]: inherited variants, which run in families, somatic mutations arising within each cell or individual, and variants that are also associated with ancient mtDNA lineages (haplogroups) and are thought to permit adaptation to changing tissue or geographic environments. High-frequency pathogenic mtDNA mutations have been found in patients with classic mitochondrial diseases, premature aging, cancers, and neurodegenerative diseases [18-23].
Mitochondrion harbors its own DNA (mtDNA), which encodes many critical proteins for the assembly and activity of mitochondrial respiratory complexes. mtDNA is packed by many proteins to form a nucleoid that uniformly distributes within the mitochondrial matrix, which is essential for mitochondrial functions. Defects or mutations of mtDNA result in a range of diseases [24]. Indeed, the small circle of mitochondrial DNA (mtDNA) present in all human cells is a rich source of pathogenic mutations and rearrangements [25].It is known that mtDNA is susceptible to environmental perturbations and that natural selection likely selected for certain mtDNA lineages [26] while selecting against less well adapted mtDNA variants. Additionally, these selective events may have produced multiple functionally important amino acid changes in ATP6, cytochrome b, and cytochrome oxidase [26].
The preservation of a functional mitochondrial genome over an individual’s lifetime requires faithful replication, segregation, and expression of appropriate function during development and in all subsequent mitotic cell divisions. Preservation also requires protection from and efficient repair of mtDNA damage [27]. It may be that genetic disease results when the number of pathogenic mtDNA mutations exceeds a certain threshold. Mitochondrial and heteroplasmy tend to show high pathogenicity, and is significantly overrepresented in disease-associated loci [16]. Purifying selection may limit the viability of these mutations and genomically standardize and lock-in specific haplotypes at the population level. This, plus the tendency toward consanguinity within ethnic groups would decrease their mtDNA genetic variability and increase within-group molecular consistency.
mtDNA variation produces increased risk of genetic disease
In the Ely-Jackson database we observed aggregations of specific mtDNA haplotypes within regionally specific ethnic groups but also unanticipated genetic variation within each of the African mitochondrial DNA (mtDNA) haplotypes expressed. Apparently most, if not all, humans contain multiple mtDNA genotypes (heteroplasmy). Specific patterns of variants accumulate in different tissues, including cancers, over time. Some molecular variants are preferentially passed down to the next generations or are suppressed in the maternal germ line [29]. This piqued our curiosity about the potential evolutionary significance of these findings. It takes energy to produce a complex mtDNA genomic environment. We anticipate that low levels of mtDNA heteroplasmy must be of evolutionary benefit, perhaps protecting against viral, fungal, or bacterial infections [30]. Whereas high levels of mtDNA mutations may increase the risk of developing common late-onset human diseases [31]. This variability within haplotypes also challenges the conventional view that high percentages of a mutation are required before a new variant has functional consequences [29].
Concentration of limited number of mtDNA haplotypes in specific human communities’ results in the aggregation of their associated genetic diseases
The population estimates of early prehistoric and historical periods are imprecise but our assumption has been that the initial populations of Homo sapiens numbered in the hundreds. Emphasis has recently focused on what was happening within the African continent before modern humans expanded their range globally [32]. Over time, the initial groups of foraging humans proliferated and at some point, early modern humans fission into smaller groups. A recent article [33] has suggested that the most plausible reticulated African population history is one in which present-day population structure dates back to 130,000 and 80,000 years ago. According to their calculations, the earliest population divergence among contemporary populations occurred 120,000 to 135,000 years ago and was preceded by links between two or more weakly differentiated ancestral Homo populations connected by gene flow over hundreds of thousands of years. As these smaller entities migrated from the original for aging regions, they may have encountered new selective forces that differently impacted on their existing genetic variation and changed the survival values associated with mutated mtDNA. Additionally, as these weakly affiliated smaller groups expanded, they moved farther from each other and the potential for regular admixture with other groups diminished. In such instances we anticipate a constriction of gene pools and a greater proportion of consanguineous reproductive mating. This would tend to concentrate the diversity of mtDNA haplogroups within the group, particularly if the in-migration of females was limited.
Since mutations in the maternally inherited mitochondrial genome (mtDNA), contribute essential protein subunits to the enzyme complexes of oxidative phosphorylation, mutations in this genome are an important cause of genetic disease. Specific mtDNA haplotypes will tend to cluster in specific ethnicgroups, it is easy to see how geospatial redundancy in mtDNA haplotypes could emerge as a precursor to ethnic and regional patterns of mtDNA-associated genetic disease that would, when previously divergent groups are reunited, influence the pattern of health disparities.
Aggregated genetic disease can display as a health disparity
Health and health care disparities refer to differences in health and health care between groups that stem from broader inequities [34-35]. A health disparity is a health difference that adversely affects disadvantaged populations in comparison to a reference population (usually in the US, upper-middle and upper-class European Americans), based on one or more health outcomes. Genetic disease can cause differential health outcomes [36]. The tricky aspect of health disparities is determining the contribution due to genetic disease. While all populations with health disparities are socially disadvantaged due in part to being subject to past racist or discriminatory actions by the larger society, they are also usually underserved in health care, thus compounding their disadvantage and reducing their survival value. Add to these volatile socio-economic circumstances the small fraction of health disparities that are due to genetic disease, and you have an amalgamation of interacting gene-environment causes that can not only amplify the extent of health disparities, it can make them seen intractable. (Figure 1) depicts the interplay of human evolution, mtDNA genetic variations, population dynamics over time, and the emergence of health disparities.
Figure 1: Depicts the interplay of human evolution, mtDNA genetic variations, population dynamics over time, and the emergence of health disparities.
Methods and Materials
Using the Ely-Jackson Database of African mtDNA variants [6] coupled with an extensive review of the scientific literature on mtDNA genetic variation and genetic disease; we researched the interrelationship between mtDNA and health disparities and have tried to place this in an evolutionary context. The databases reviewed included the following:
- AmtDB (https://amtdb.org/)
- GenBank (https://isogg.org/wiki/GenBank)
- Helix mitochondrial DNA database (https://www.helix.com/pages/mitochondrial-variant-database)
- mtDB [37]
- MSeq DR Consortium (https://mseqdr.org/)
- Ely-Jackson Database of African and African American mtDNA variants
The Ely-Jackson Database is a specialized, expertly curated database with information on sequenced HVS1 and HVS2, detailed ethnographic, and geospatial information on all 4291 samples. Named after senior biologists Bert Ely (University of South Carolina) and the late Bruce Jackson (University of Lowell), the database was collected in the early 2000s and was used initially to determine the number of African ethnic groups that matched the mtDNA haplotypes observed in African Americans [6]. Since then, we have developed a phylogenetic tree of African American mtDNA variants linked to specific African ethnic and regional groups. We also developed an inclusive phylogenetic tree of African mtDNA variants associated with specific African linguistic, ethnographic, and geospatial groups. These efforts familiarized us with the range of molecular variation in mtDNA and the phylogenetic relationships between the different haplotypes.
In the Ely-Jackson database we observed unanticipated genetic variation within each of the 85African mitochondrial DNA (mtDNA) haplotypes. Apparently most, if not all, humans contain multiple mtDNA genotypes (heteroplasmy) and specific patterns of variants accumulate in different tissues, including cancers, over time. Some of these molecular variants are preferentially passed down or suppressed in the maternal germ line [29]. This piqued our curiosity about the evolutionary significance of these findings. While these findings suggest that there must be a benefit cast light on the origin and spread of mtDNA mutations at multiple scales, from the organelle to the human population, and challenge the conventional view that high percentages of a mutation are required before a new variant has functional consequences.
For this report, the published scientific literature was comprehensively search for mtDNA variants known to be correlated with specific types of genetic disease. These databases included:
- PubMed PubMed(https://pubmed.ncbi.nlm.nih.gov/)
- Embase (https://www.elsevier.com/solutions/embase-biomedical-research)
- LILACS (https://lilacs.bvsalud.org/en/)
- ScienceDirect (https://www.sciencedirect.com/)
- GoogleScholar (https://scholar.google.com)
Bibliometric searches were conducted using these reference databases using the following keywords: mtDNA, genetic disease, evolution, physiology, and health disparities. The resulting documents were then organized by presumed genetic disease association and/or by mtDNA haplotype. A table was created (Table 1) that summarizes the core data needed to recognize the depth and breadth of the numerous associations between mtDNA and specific genetic diseases.
Results
mtDNA Haplotype | Associated Genetic Disease | Physiological Consequences of mtDNA variant on genetic disease | Effects of mtDNA variant on genetic disease | Affected Population | Reference |
A | T2DM | Unknown | RIsk | Meta | -45 |
A | AIDS susceptibility | Possible differences in max. temp, ROS protection | Risk | Han Chinese, Southwestern China | -51 |
A | MS | ROS | Risk | Iranian Persian | -52 |
A | atherothrombotic cerebral infarction | Unknown | Risk | Japanese Females | -53 |
B | Knee Osteoarthritis | Unknown, but mitochondria play a critical role in bone homeostasis | Risk | Korean | -84 |
B4 | Oral lichen planus | Unknown | Protection | Chinese | -54 |
B4b′d′e′j | Gallstone disease | Unknown | Risk | Shanghainese | -55 |
B5 | Alzheimer's | Increased ROS | Risk | Southern and East Chinese | -56 |
D | End stage renal failure, early onset | Unknown | Risk | Han Chinese | -57 |
D | HTN | Unknown | Risk | Han Chinese | -57 |
D | Low Total Cholesterol | Unknown | Risk | Han Chinese | -57 |
D | Low Total Cholesterol (Young) | Unknown | Risk | Han Chinese | -57 |
D | Lung CA | Unknown | Protection | Han Chinese, Southwestern China | -57 |
D4a | Thyroid CA | Unknown | Risk | Wenzhou, China | -58 |
D4 | AML | Unknown | Risk | Korean | -59 |
M (Subgroup D5) | Breast CA | Unknown | Risk | Wenzhou, China | -58 |
D5 (SuperGroupM) | T2DM | Unknown | Protection | Taiwan | -60 |
D5 (Supergroup N) | T2DM | Unknown | Protection | Taiwan | -60 |
F | Lung CAm | Unknown | Protection | Han Chinese, Southwestern China | -57 |
F | T2DM | Unknown | Risk | Meta | -45 |
F4 (SupergroupM) | T2DM | Unknown | Protection | Taiwan | -60 |
F4 (SupergroupN) | T2DM | Unknown | Protection | Taiwan | -60 |
F4 (D5) | HTN | Increased ROS | Risk | Taiwan | -60 |
F4 (Supergroup M) | HTN | Increased ROS | Risk | Taiwan | -60 |
F4 (Super group N) | HTN | Increased ROS | Risk | Taiwan | -60 |
F4 (D5) | Obesity | Unknown, but mitochondria control conversion of adipose into brown fat | Risk | Taiwan | -60 |
F4 (Macro group M) | Obesity | Unknown, but mitochondria control conversion of adipose into brown fat | Risk | Taiwan | -60 |
F4 (Macrgroup N) | Obesity | Unknown, but mitochondria control conversion of adipose into brown fat | Risk | Taiwan | -60 |
G | Lung CA | Unknown | Risk | Han Chinese, Southwestern China | -57 |
H | Alzheimer's | Elevated ROS | Risk of Disease | Meta Analysis, mostly European; Poland | (45); (61); (62) |
H | LHON-associated visual failure | Unknown | Protection | European | -63 |
H5 | Alzheimer's | Increased ROS (recall heterogeneity in J) | Risk | Northern + Southern Italy | -64 |
H5 (synergy with APOE 4) | Alzheimer's | Unknown | Risk | Poland | -62 |
H5, in the presence of APOE 4 | Alzheimer's | Increased ROS (recall heterogeneity in J) | Risk | Northern + Southern Italy | -64 |
H5a | Alzheimer's | Increased ROS (recall heterogeneity in J) | Risk | Northern + Southern Italy | -64 |
H6A1A | Alzheimer's | Unknown | Protection | Utah (Cache County Cohort) | -65 |
H6A1B | Alzheimer's | Unknown | Protection | Utah (Cache County Cohort) | -9 |
HV | Schizophrenia | Increased ROS | Risk of Disease | Israeli Arabs | -66 |
HV | Alzheimer's | Increased ROS (recall heterogeneity in J) | Risk | Poland | -62 |
HV | Parkinson’s | Elevated ROS | Risk | Meta Analysis, mostly European | -45 |
Pre-HV | Bipolar (unspecified type) and schizophrenia | Possible Coupling Differences | Risk | U.S. citizens | -67 |
J | Parkinson’s | Decreased ROS | Protection | Meta Analysis, mostly European; U.S. Caucasians | -45 |
J | 3MS score decline (8-year period) | High ROS for some subtypes | Risk | Memphis and Pittsburg | -68 |
J | Alzheimer's | Unknown | Protection | Poland | -62 |
J | Longevity | ROS | Associated | Meta | -45 |
J | LHON-associated visual failure | Unknown | Risk | European | -63 |
J1 (Compared to J2) | LHON-associated visual failure | Unknown | Risk | European | -63 |
J2 (compared to J1) | LHON-associated visual failure | Unknown | Risk | European | -63 |
J1b | Parkinson’s | Decreased ROS | Protection | UK | -63 |
JK | Parkinson’s | Decreased ROS | Protection | U.S. Caucasians | -69 |
JT | Parkinson’s | Decreased ROS | Protection | UK | -63 |
JT | Alzheimer's | Reduced ROS, possibly higher | Protection | Poland | -62 |
activity of catalases | |||||
JT | Schizophrenia | Unknown, recall heterogeneity in J | Risk of early onset of disease | Italy | -70 |
JT | Parkinson’s | Decreased ROS | Protection | Meta | -63 |
UKJT | Parkinson’s | Unknown | Delayed onset | Cypriot Greek | -71 |
UKJT | Alzheimer's | Decreased ROS | Protection | Western Europe | -72 |
K | Alzheimer's | Unknown | Protection—e specially from APOE4 | Poland | -62 |
K | Schizophrenia | Unknown | Risk | European, UK | -66 |
K | Parkinsons | Decreased ROS | Protection | Meta Analysis, mostly European; U.S. Caucasians | (45); (69); (61) |
K | Breast Cancer | Unknown | Risk | North American European | -73 |
K | Breast CA | Unknown | Risk | European American | -74 |
K | MS | ROS | Risk | Iranian Persian | -52 |
K | LHON-associated visual failure | Unknown | Risk | European | -63 |
K1 | Childhood Acute Lymphoblastoid Leukemia | Unknown | Risk | Finland | -75 |
K1A1B and K1A1B2A1 | Decreased Temporal Pole Thickness | Unknown | Risk | US + Canada | -65 |
KU | Alzheimer's | Unknown | Protection | Poland | -62 |
L | Colorectal Cancer | Unknown | Risk | Multi ethnic cohort from Hawaii and California | -88 |
L1 | Alzheimer's | Unknown | Risk | African Americans | -68 |
LMN | Parkinson’s | Unknown | Protection | Cypriot Greek | -71 |
M | Breast CA | Unknown | Risk | Wenzhou, China | -58 |
M7 | Lung CA | Unknown | Risk | Han Chinese, Southwestern China | -57 |
N9a (Super | HTN | Increased ROS | Risk | Taiwan | -60 |
group N) | |||||
N9a | Bipolar (unspecified type) | Unknown | Risk | Japan | -38 |
N9a | Schizophrenia | Unknown | Risk | European, UK | -63 |
N9a | T2DM | Unknown | Protection | Asians | (76);(60) |
N1a1 | Schizophrenia | Unknown | Risk | European, UK | -63 |
NWXI | Parkinson’s | Unknown | Protection | Cypriot Greek | -71 |
T | Parkinson’s | Decreased ROS, better response to oxidation shock | Protection | Meta Analysis, mostly European | (45); (61) |
T | Dementia | Unknown | Risk | Pittsburg | -68 |
T | Alzheimer's | Decreased ROS | Protection | Poland | -62 |
T | Bipolar (unspecified type) and schizophrenia | Possible Coupling Differences | Protection | U.S. citizens | -67 |
T | Colorectal Cancer | Unknown | Risk | Multi ethnic cohort from Hawaii and California | -88 |
T | T2DM | Unknown | Risk | Asians | -76 |
U | Bipolar- Associated Psychosis | Unknown | Risk | European, UK | -77 |
U | T2DM Vascular complications | Unknown | Protection | Finland | -78 |
U | T2DM maternal family history | Unknown | Association | Finland | -78 |
U | Breast CA | Unknown | Protection | European American | -74 |
U | Breast Cancer | Unknown | Protection | North American Caucasian | -73 |
U | Prostate Cancer | Unknown | Risk | North American Caucasian | -79 |
U | Renal Cancer | Unknown | Risk | North American Caucasian | -79 |
U | Atherosclerosis | ROS | Risk | Polish | -80 |
U5 | Schizophrenia | Unknown | Risk | European, UK | -31 |
U9 | Schizophrenia | Unknown | Risk | European, UK | -31 |
U5a | Schizophrenia | Unknown | Risk | European, UK | -31 |
U5a1 | Schizophrenia | Unknown | Risk | European, UK | -31 |
U5B1 or U5B1B2 | Decreased Temperal Pole Thickness | Unknown | Risk | US + Canada | -65 |
V | T2DM | Unknown | Protection | Polish | -80 |
W | Longevity | ROS | Not Associated | Meta | -45 |
Branch 155 | Decreased Whole Brain volume | Unknown | Risk | US + Canada | -83 |
Branch 184 | Decreased Whole Brain Volume | Unknown | Risk | US + Canada | -83 |
Branch 184 | Left Hippocampal Atrophy | Unknown | Risk | US + Canada | -65 |
p.G166G; m.15244A>G | Decline in DSST score | Unknown | Risk | Memphis and Pittsburg | -68 |
p.I166V; m.14178T> C | 3MS score decline (8-year period) | Unknown | Risk | Memphis and Pittsburg | -68 |
Disagreeing Studies | Alzheimer's, Parkinson’s, migriane | NONE | NONE | Spanish | (81); (82) |
Table 1: A cross-section of articles from the published scientific literature detailing the associations of mtDNA haplotypes and genetic disease.
Discussion
Encoding only 37 genes, mitochondria have evolved to become an essential organelle within the cell [31]. The mitochondrion’s compact structure and minimal redundancy results in mutations on the mitochondrial genome being an important cause of genetic disease. Our search of the literature revealed numerous associations between mtDNA variants and genetic disease with many of these diseases displaying important health disparities.
The comprehensive literature search results displayed in Table 1show that the numbers of risk association variants (N=59) are much greater than the number of protective associations variants (N=33). This is likely a reflection of our pathology driven biomedical research paradigm [39]. Abnormalities are more likely to gain the attention of the clinical community and Big Pharma than are mtDNA variants that are deterrents to genetic disease. In spite of the literature’s emphasis on disease risk alleles, very few explicit physiological consequences of specific variants have been identified in the literature. This likely reflects the still early stage of the research on the associations of specific mtDNA types and particular genetic diseases.
Our survey suggests that only 3 out of 95 entries are from megahaplo group L, even thought this is the oldest haplogroup in our species and could, therefore, provide the greatest evolutionary information about the complex association of mtDNA variants, genetic disease, and current health disparities. Low coverage of "megahaplo group” L is consistent with the general underrepresentation in the scientific literature of studies on genetic variants more commonly observed in peoples of recent African descent. While there have been many calls for parity in research [40-41],this has not yet translated into adequate representation of African mtDNA variants in either disease association studies or cell culture experiments [42].In one of the few studies of molecular and bioenergetic differences between regionally diverse mtDNA haplogroups [43] the L "megahaplo group”, common in peoples of recent African descent, showed lower mtDNA copy numbers, but higher expression levels for nine mtDNA-encoded respiratory complex genes, decreased ATP (adenosine triphosphate) turnover rates and lower levels of reactive oxygen species production, parameters which were considered consistent with more efficient oxidative phosphorylation. In a more recent study, comparisons between "megahaplo group” L and haplogroup H (common in Europeans) showed important physiological differences including variations in (i) responses to exogenous stressors (Amyβ and UV radiation), (ii) epigenetic status, and (iii) modulation profiles of methylation-mediated downstream complement, inflammation, and angiogenesis genes [44]. These latter differences are commonly associated with various human diseases, many of which display important health disparities.
In our study, we identified 29 clinical conditions associated with mtDNA mutations. The most commonly encounter pathologies were cancer, neurodegenerative diseases, and metabolic disorders. The cancers included lung, thyroid, breast, lymphoblastic, and colorectal. Several mutations in nuclear genes encoding for mitochondrial components have been associated with an increased cancer risk [23]. Previous studies have associated a range of mtDNA abnormalities, including mutations, deletions, inversions and copy number alterations, with mitochondrial dysfunction and these changes may be the biogenesis of hampered cellular bioenergetics in diverse cancer cell types [23].
Neurodegenerative diseases were a second target of mtDNA dysfunction. These include Alzheimer’s, Schizophrenia, Parkinson’s, bipolar, MS, Leber hereditary optic neuropathy, and dementia. Several symptoms were reported that may be precursors to these neurodegenerative malfunctions, including left hippocampus atrophy, decreased temporal pole thickness, decreased whole brain volume, migraine, and declines in performance on the digital symbol substitution test. Mitochondrial dysfunction is directly implicated in several neurodegenerative diseases, and is potentially related to increased oxidative damage and amyloid-β (Aβ) formation in Alzheimer's disease [46].
Metabolic disorders reported in our search of the literature included reports of Type 2 diabetes mellitus, gallstone disease, low total cholesterol, and obesity. It has been reported that European mtDNA haplogroups may play an important role in susceptibility to metabolic disturbance and cardiovascular disease [47,48]. It is likely that our lists of disruptive mtDNA genetic disease conditions (metabolic, carcinogenic, and neurodegenerative) are largely a reflection of the sampled base populations. This presents a dilemma for making solid correlations to existing health disparities.
In the scientific literature, the populations sampled for mtDNA genetic disease associations are in inverse proportion to the patterns of current health disparities. The US groups with persistently disproportionate health disparities include African Americans/Blacks, American Indians and Alaska Natives, Asians, Native Hawaiians/other Pacific Islanders and Hispanics/Latinos. The literature focused 59 out 95 cases (62%) in Europeans and peoples of European descent although worldwide, Europeans are estimated to comprise only 12% of the world’s population. There were 32/95 cases (34%) in the literature that considered Asians although Asia represents 30% of the world’s land mass and over 60% of the world’s current population are Asians. In our review of the literature, the overwhelming majority of the represented Asians were East Asians. The mtDNA genetic disease associations in peoples of African descent represented only 1% of all studies we reviewed. "YetAfrica" is the second most populous continent in the world and there are approximately 150 million African-descended people worldwide, according to the United Nations. Additionally, the high genetic diversity observed in peoples of recent African descent and the antiquity of the human presence in continental Africa, mandates that more than 1% of our scientific effort be focused on the disease consequences of molecular variation observed in these populations.
To increase parity in genetic disease studies, we must intentionally collect from the underrepresented populations using big data storage, processing, exchange and curation [87]. Once researchers commit to including more diversity in mtDNA genetic disease studies, increasing the bibliometric databases to include, for example, Web of Science (WoS), Google Scholar, Microsoft Academic, Crossreff, Dimensions and Cite Seer [49] will enhance the catchment area of relevant publications. Right now, however, we are at the beginning of understanding the complex relationships of mtDNA haplotypes, genetic disease incidence, and the contribution to health disparities. Since mtDNA mutations may cause unanticipated, extended phenotypes and have reproductive implications [50] it is imperative that we systematically broaden the sampling database of these studies so that the evolutionary and biomedical inferences can be anticipated.
Competing Interests
The authors declare no competing interests.
Author Credits
The manuscript was initiated, figure 1 conceived, and the text was written by FJ. Table 1 was compiled by LM with input from JC, NB, MM, and KK. All authors collaborated on the final manuscript.
Acknowledgements
We thank the All of Us Researcher Academy and RTI for support of this study and the QuadGrid Data Lab Research Group for bibliometric assistance throughout the project. We thank Dr. Bert Ely, University of South Carolina for access to the Ely-Jackson Database of African and African American mtDNA variants. This work is supported by the Division of Engagement and Outreach, All of Us Research Program, National Institutes of Health under award number 1OT20D028395-01."
References
- Salas A, Richards M, De la Fe T, Lareu MV, Sobrino B, et al. (2002) The Making of the African mtDNA Landscape. Am J Hum Genet. 71(5):1082-11.
- Gonder MK, Mortensen HM, Reed FA, de Sousa A. (2007) Whole-mtDNA Genome Sequence Analysis of Ancient African Lineages. Mol Biol Evol. 24(3):757–68.
- Chen YS, Oickers A, Schurr TG, Kogelnik AM, Huopenen K, et al. (2000) mtDNA Variation in the South African Kung and Khwe—and Their Genetic Relationships to Other African Populations. Am J Hum Genet. 66(4):1362-83.
- Forster P. (2004) Ice Ages and the mitochondrial DNA chronology of human dispersals: a review. Philos Trans R Soc Lond B Biol Sci. 359(1442):255-64.
- Rosa A, Brehem A. (2011) African human mtDNA phylogeography at-a-glance. J Anthropol Sci. 89:25-58.
- Ely B, Wilson JL, Jackson F, Jackson BA. (2006) African-American mitochondrial DNAs often match mtDNAs found in multiple African ethnic groups. BMC biology. 4(1):1-14.
- Beyer RM, Krapp M, Manica A. (2020) High-resolution terrestrial climate, bioclimate and vegetation for the last 120,000 years. Sci Data. 7 (1):236.
- Leplongeon A. ( 2021) The Main Nile Valley at the End of the Pleistocene (28–15 ka): Dispersal Corridor or Environmental Refugium? Front. Earth Sci Sec. 1-22.
- Ridge PG, Maxwell TJ, Corcoran CD, Norton MC, Tschanz J.T, et al. (2012) Mitochondrial genomic analysis of late onset Alzheimer’s disease reveals protective haplogroups H6A1A/h6a1b: The Cache County Study on memory in aging. PLoS ONE, 7(9):e45134.
- Stojanowski CM, Carver CL, Miller KA. (2014) Incisor avulsion, social identity and Saharan population history: New data from the Early Holocene southern Sahara. J Anthropol Archaeol. 35:79-91.
- Menocal PB de. (2015) Palaeoclimate: End of the African Humid Period. Nat Geosci. 8(2):86–87.
- Černý V, Hajek M, Bromova M, Čmejla R, Diallo I, et al. (2006) MtDNA of Fulani nomads and their genetic relationships to neighboring sedentary populations. Hum Biol. 78(1): 9-27..
- Behar DM, Villems R, Soodyall H, Blue-Smith J, Pereira L, et al. (2008) The Dawn of Human Matrilineal Diversity. Am J Hum G. 82(5):1130-40.
- Watson E, Bauer K, Aman R, Weiss G, von Haeseler A, et al. (1996) mtDNA sequence diversity in Africa. Am J Hum Genet. 59(2):437-44.
- Lipson M, Sawchuk EA, Thompson JC, Oppenheimer J, Tryon CA, et al. (2022) Ancient DNA and deep population structure in sub-Saharan African foragers. Nature. 603:290–96.
- Ye K, Lu J, Ma F, Gu Z. (2014) Extensive pathogenicity of mitochondrial heteroplasmy in healthy human individuals. PLOS One. 111(29):10654-659.
- Kopinski PK, Singh LN, Zhang S, Lott MT, Wallace DC. (2021) Mitochondrial DNA variation and cancer. Nat Rev Cancer. 21:431–45.
- Disotell TR. (2003) Discovering human history from stomach bacteria. Genome Biol. 4(5):213. https://doi.org/10.1186/gb-2003-4-5-213
- Salminen TS, Vale PF. (2020) Drosophila as a Model System to Investigate the Effects of Mitochondrial Variation on Innate Immunity. Front Immunol. 11:521.
- Wu J, Ma Z, Raman A, Beckerman P, Dhillon P, et al. (2021) APOL1 risk variants in individuals of African genetic ancestry drive endothelial cell defects that exacerbate sepsis. Immunity. 54(11):2632:49.
- Keogh MJ, Chinnery PF. (2015) Mitochondrial DNA mutations in neurodegeneration. Biochimica Biophysica Acta. 1847 (11):1401-11.
- Scotece M, Vaamonde-García C, Lechuga-Vieco AV, Cortés AC, Gómez MCJ, et al. mtDNA variability determines spontaneous joint aging damage in a conplastic mouse model. Aging (Albany NY). 14(15):5966:83.
- Van Gisbergen MW, Voets AM, Starmans MHW, de Coo IFM, Yadak R, et al. (2015) How do changes in the mtDNA and mitochondrial dysfunction influence cancer and cancer therapy? Challenges, opportunities and models. Mutat Res Rev Mutat Res. 764:16-30.
- Yan C, Duanmu X, Zeng L, Liu B, Song Z. (2019) Mitochondrial DNA: distribution, mutations, and elimination. Cells. 8(4):379.
- DiMauro S, Davidzon G. (2009) Mitochondrial DNA and disease. Annals of Medicine. 37(3):2-11.
- Mishmar D, Ruiz-Pesini E, Golik P, Macaulay V, Clark AG, et al (2003) Natural selection shaped regional mtDNA variation in humans. Proc Natl Acad U S A. 100(1):171-76.
- Shadel GS. (1999) Yeast as a Model for Human mtDNA Replication. Am J Hum Genetic 65(5):1230-37.
- Li Y, Beckman KB, Caberto C, Kazma R, Lum-Jones A, et al. (2015) Association of Genes, Pathways, and Haplogroups of the Mitochondrial Genome with the Risk of Colorectal Cancer: The Multiethnic Cohort. PLOS ONE, 10(9):e0136796.
- Stewart JB, Chinnery PF. (2021) Extreme heterogeneity of human mitochondrial DNA from organelles to populations. Nat Rev Genet 22(2):106–18.
- Saffran HA, Pare JM, Corcoran JA, Weiller SK, Smiley JR. (20 07) Herpes simplex virus eliminates host mitochondrial DNA. EMBO Reports. 8(2):188-93.
- Hudson G, Gomez-Duran A, Wilson IJ, Chinnery PF. (2014) Recent mitochondrial DNA mutations increase the risk of developing common late-onset human diseases. PLOS Genetics 10(5): e1004369.
- Henn BM, Steele TE, Weaver TD. (2018) Clarifying distinct models of modern human origins in Africa. Curr Opin Genet Dev. 53:148-56.
- Ragsdale AP, Weaver TD, Atkinson EG, Hoal EG, Moller M, et al. (2023) A weakly structured stem for human origins in Africa. Nature. 617(7962):755–63.
- https://www.kff.org/racial-equity-and-health-policy/issue-brief/disparities-in-health-and-health-care-5-key-question-and-answers/#:~:text=A%20%E2%80%9Chealth%20disparity%E2%80%9D%20refers%20to%20a%20higher%20burden,and%20use%20of%20care%2C%20and%20quality%20of%20care
- https://www.verywellhealth.com/health-disparities-4173220
- Kim MS, Patel KP, Teng AK, Berens AJ, Lachance J. (2018) Genetic disease risks can be misestimated across global populations. Genome Biol. 19(1):1-14.
- Ingman M, Gyllensten U. (2006) mtDB: Human Mitochondrial Genome Database, a resource for population genetics and medical sciences. Nucleic Acids Res. 34(database issue):D749-51.
- Kazuno A, Munakata K, Mori K, Nanko S, Kunugi H, et al. (2009) Mitochondrial DNA haplogroup analysis in patients with bipolar disorder. Am J Med Genet Neuropsychiatr Genet. 150B(2):243–47.
- Keating P, Cambrosio A. (2004) Does Biomedicine Entail the Successful Reduction of Pathology to Biology? Perspect Biol Med. 47(3):357-71.
- Deyrup A, Graves JL Jr. (2022) Racial Biology and Medical Misconceptions. N Engl J Med. 386(6):501-3.
- Clark US, Hurd YL. (2020) Addressing racism and disparities in the biomedical sciences. Nat Hum Behav. 4(8):774–77.
- Suissa S, Wang Z, Poole J, Wittkopp S, Feder J, Shutt et al. (2009) Ancient mtDNA genetic variants modulate mtDNA transcription and replication. PLoS Genet. 5(5):e1000474.
- Kenney MC, Chwa M, Atilano SR, Falatoonzadeh P, Ramirez C, et al. (2014) Molecular and bioenergetic differences between cells with African versus European inherited mitochondrial DNA haplogroups: implications for population susceptibility to diseases. Biochim Biophys Acta. 1842(2):208-19.
- Amar S, Shamir A, Ovadia O, Blanaru M, Reshef A, et al. (2007) Mitochondrial DNA HV lineage increases the susceptibility to schizophrenia among Israeli Arabs. Schizophr Rese. 94(1-3):354-58.
- Marom S, Friger M, Mishmar D. (2017) MtDNA meta-analysis reveals both phenotype specificity and allele heterogeneity: A model for differential association. Sci Rep. 7(1):1-11.
- Tranah TJ, Yokoyama JS, Katzman SM, Nails MA, Newman AB, et al. (2014) Mitochondrial DNA sequence associations with dementia and amyloid-β in elderly African Americans. Neurobiol Aging. 35(2):442.e1-8.
- Micheloud D, Berenguer J, Guzmán-Fulgencio M, Campos Y, García-Álvarez M, et al. (2011) European Mitochondrial DNA Haplogroups and Metabolic Disorders in HIV/HCV-Coinfected Patients on Highly Active Antiretroviral Therapy. J Acquir Immune Defic Syndr. 58(4):371-78.
- Suomalainen A, Pirjo I. (2010) Mitochondrial DNA depletion syndromes – Many genes, common mechanisms. Neuromuscul Disord. 20(7):429-37.
- de Araujo LS, Ribeiro-Alves M, Leal-Calvo T, Leung J, Durán V, et al. (2019) Reprogramming of Small Noncoding RNA Populations in Peripheral Blood Reveals Host Biomarkers for Latent and Active Mycobacterium tuberculosis Infection. mBio. 10(6):e01037-19.
- Macken WL, Lucassen AM, Hanna MG, Pitceathly RDS. (2021) Mitochondrial DNA variants in genomic data: diagnostic uplifts and predictive implications. Nat Rev Genet 22(9):547–48.
- Wang HW, Xu Y, Miao YL, Luo HY, Wang KH. (2014) Mitochondrial DNA haplogroup a May confer a genetic susceptibility to AIDS group from Southwest China. Mitochondrial DNA A DNA A DNA Mapp seq Anal. 27(3):1–4.
- Kumleh HH, Houshmand M, Panahi MS, Riazi GH, Sanati MH, et al. (2006) Mitochondrial D-loop variation in Persian multiple sclerosis patients: K and a haplogroups as a risk factor!!. Cell Mol Neurobiol. 26(2):119–25.
- Nishigaki Y, Yamada Y, Fuku N, Matsuo H, Segawa T, et al. (2007) Mitochondrial haplogroup A is a genetic risk factor for atherothrombotic cerebral infarction in Japanese females. Mitochondrion. 7(1-2), 72-79.
- Wu D, Cheng S, Chen X, Sun M, Wang G, et al. (2013). Mitochondrial haplogroup B4 may be a protective factor to oral lichen planus susceptibility in Chinese. Oral Dis. 20(1):62–8.
- Sun D, Niu Z, Zheng H, Wu F, Jiang L, et al. (2021) A Mitochondrial DNA Variant Elevates the Risk of Gallstone Disease by Altering Mitochondrial Function. Cell Mol Gastroenterol Hepatol. 11(4):1211-26.
- Bi R, Zhang W, Yu D, Li X, Wang HZ, et al. (2015) Mitochondrial DNA haplogroup B5 confers genetic susceptibility to Alzheimer’s disease in Han Chinese. Neurobiol Aging. 36(3):1604.e7-e16.
- Zhang Y, Zhao Y, Wen S, Yan R, Yang Q, et al. (2016) Associations of mitochondrial haplogroups and mitochondrial DNA copy numbers with end-stage renal disease in a Han population. Mitochondrial DNA A DNA Mapp Seq Anal. 28(5):725–31.
- Fang H, Shen L, Chen T, He J, Ding Z, et al. (2009) Cancer type-specific modulation of mitochondrial haplogroups in breast, colorectal and thyroid cancer. BMC Cancer. 10: 421.
- Kim HR, Kang MG, Lee YE, Na BR, Noh MS, et al. (2018) Spectrum of mitochondrial genome instability and implication of mitochondrial haplogroups in Korean patients with acute myeloid leukemia. Blood Res. 53(3):240-49.
- Chalkia D, Chang Y, Derbeneva O, Lvova M, Wang P, et al. (2018) Mitochondrial DNA associations with East Asian metabolic syndrome. Biochim Biophys Acta Bioenerg. 1859(9):878-92.
- Ghezzi D, Marelli C, Achilli A, Goldwurm S, Pezzoli G, et al. (2005) Mitochondrial DNA haplogroup K is associated with a lower risk of Parkinson's disease in Italians. Eur J Hum Genet. 13(6):748-52.
- Maruszak A, Safranow K, Branicki W, Gawęda-Walerych K, Pośpiech E, et al. (2011) The impact of mitochondrial and nuclear DNA variants on late-onset Alzheimer’s disease risk. J Alzheimer’s Dis. 27(1):197–10.
- Hudson G, Nalls M, Evans JR, Breen DP, Winder-Rhodes S, et al. (2013) Two-stage association study and meta-analysis of mitochondrial DNA variants in Parkinson disease. Neurology. 80(22):2042-48.
- Santoro A, Balbi V, Balducci E, Pirazzini C, Rosini F, et al. (2010) Evidence for sub-haplogroup H5 of mitochondrial DNA as a risk factor for late onset alzheimer’s disease. PLoSONE. 5(8):e12037.
- Ridge PG, Koop A, Maxwell TJ, Bailey MH, Swerdlow RH, et al. (2013) Mitochondrial haplotypes associated with biomarkers for Alzheimer’s disease. PLoS One. 8(9):e74158.
- Hudson G, Gomez-Duran A, Wilson IJ, Chinnery PF. (2014) Recent mitochondrial DNA mutations increase the risk of developing common late-onset human diseases. PLoS genetics. 10(5):e1004369.
- Rollins B, Martin MV, Sequeira PA, Moon EA, Morgan LZ, et al. (2008) Mitochondrial Variants in Schizophrenia, Bipolar Disorder, and Major Depressive Disorder. PLoS ONE. 4(3):e4913.
- Tranah GJ, Nalls MA, Katzman SM, Yokoyama JS, Lam ET, et al. (2011) Mitochondrial DNA Sequence Variation Associated with Dementia and Cognitive Function in the Elderly. J Alzheimers Dis. 32(2):357-72.
- Van der Walt JM, Nicodemus KK, Martin ER, Scott WK, Nance MA, et al. (2003) Mitochondrial Polymorphisms Significantly Reduce the Risk of Parkinson Disease. Am J Hum Genet. 2(4):804-11.
- Magri, C., Gardella, R., Barlati, S. D., Valsecchi, P., Sacchetti, E., &Barlati, S. (2007). Mitochondrial DNA haplogroups and age at onset of schizophrenia. American Journal of Medical Genetics Part B: Neuropsychiatric Genetics, 144B(4), 496–501. https://doi.org/10.1002/ajmg.b.30496
- Georgiou A, Demetriou CA, Heraclides A, Christou YP, Leonidou E, et al. (2017) Mitochondrial superclusters influence age of onset of Parkinson’s disease in a gender specific manner in the Cypriot population: A case-control study. PLoS One. 12(9) :e0183444.
- Papapetropoulos S. (2007) Mitochondrial DNA haplogroup cluster UKJT reduces the risk of PD. Yearbook of Neurosurgery. 187–88.
- Ryu E, Nassan M, Jenkins GD, Armasu SM, Andreazza A,et al. (2018) A genome-wide search for bipolar disorder risk loci modified by mitochondrial genome variation. Mol Neuropsychiatry. 3(3):125–34.
- Bai RK, Leal SM, Covarrubias D, Liu A, Wong LJC. (2007) Mitochondrial genetic background modifies breast cancer risk. Cancer Res. 67(10): 4687–94.
- Järviaho T, Hurme-Niiranen A, Soini HK, Niinimäki R, Möttönen M, et al. (2017) Novel non-neutral mitochondrial DNA mutations found in childhood acute lymphoblastic leukemia. Clin Genet. 93(2):275–85.
- Fuku N, Park KS, Yamada Y, Nishigaki Y, Cho YM,et al. (2007) Mitochondrial Haplogroup N9a Confers Resistance against Type 2 Diabetes in Asians. Am J Hum Genet. 80(3):407-15.
- Frye MA, Ryu E, Nassan M, Jenkins GD, Andreazza AC,et al. (2017) Mitochondrial DNA sequence data reveals association of haplogroup U with psychosis in bipolar disorder. J Psychiatr Res. 84:221–26.
- Martikainen MH, Rönnemaa T, Majamaa K. (2015) Association of mitochondrial DNA haplogroups and vascular complications of diabetes mellitus: A population-based study. Diab Vasc Dis Res. 12(4):302-4.
- Booker LM, Habermacher GM, Jessie BC, Sun QC, et al. (2006) North American white mitochondrial haplogroups in prostate and renal cancer. J Urol. 175(2):468–73.
- ElsonJL, Piwonska A, Drygas W, Ploski R, Bartnik E, et al. (2019) New mtDNA Association Model, MutPred Variant Load, Suggests Individuals With Multiple Mildly Deleterious mtDNA Variants Are More Likely to Suffer From Atherosclerosis. Front Genet. 9:702.
- Fachal L, Mosquera-Miguel A, Pastor P, Ortega-Cubero S, Lorenzo E, et al. (2014) No evidence of association between common European mitochondrial DNA variants in Alzheimer, Parkinson, and migraine in the Spanish population. American Journal of Medical Genetics Part B: Neuropsychiatric Genetics. 168(1): 54–65.
- Huerta C, Castro MG, Coto E, Blázquez M, Ribacoba R, et al. (2005) Mitochondrial DNA polymorphisms and risk of Parkinson's disease in Spanish population. JNeurol Sci. 236(1-2):49-54.
- Hudson G, Gomez-Duran A, Wilson IJ, Chinnery PF. (2014) Recent mitochondrial DNA mutations increase the risk of developing common late-onset human diseases. PLOS Genetics 10(5): e1004369.
- Koo BS, SongY, Lee S, Sung K, Shin J, et al. (2019) Association of Asian mitochondrial DNA haplogroup B with new development of knee osteoarthritis in Koreans. Int J Rheum Dis.22(3):411-416.
- Renssen H, Brovkin V, Fichefet T, Goosse H. (2006) "Simulation of the Holocene climate evolution in Northern Africa: The termination of the African Humid Period". Quaternary International. 150 (1): 95.
- Liu X, Rendle-Bühring R, Kuhlmann H, Li A. (2017) Two phases of the Holocene East African Humid Period: Inferred from a high-resolution geochemical record off Tanzania. Earth and Planetary Science Letters. 460:123–134.
- Zou D, Ma L, Yu J, Zhang Z. (2015) Biological databases for human research. Genomics Proteomics Bioinformatics.13(1):55-63.
- Li M, Schröder R, Ni S, Madea B, Stoneking M. (2015) Extensive tissue-related and allele-related mtDNAheteroplasmy suggests positive selection for somatic mutations. Proceedings of the National Academy of Sciences. 112(8), 2491-96.
- Vigilant L, Stoneking M, Harpending H, Hawkes K, Wilson AC. (1991) African Populations and the Evolution of Human Mitochondrial DNA Science. Science. 253(5027):1503-07.
- Howell N, Elson JL, Turnbull DM, Herrnstadt C. (2004) African Haplogroup L mtDNA Sequences Show Violations of Clock-like Evolution. Mol BiolEvol. 21(10):1843-54.
- Allard RW. (1975) The mating system and microevolution. Genetics.79 Suppl:115-26.
- Williams-Blangero S. (1990) Population structure of the Jirels: patterns of mate choice. Am J Phys Anthropol. 82(1):61-71.
- Conroy-Beam D, Buss DM, Pham MN, Shackelford TK. (2015) How Sexually Dimorphic Are Human Mate Preferences. Pers Soc Psychol Bull. 41(8):1082-93.
- Mishmar D, Ruiz-Pesini E, Golik P, Macaulay V, Clark AG, et al. (2003) Natural selection shaped regional mtDNA variation in humans. Proc Natl Acad Sci U S A. 100(1):171-6.
- Ruiz-Pesini E, Mishmar D, Brandon M, Procaccio V, Wallace DC. (2004) Effects of purifying and adaptive selection on regional variation in human mtDNA. Science.303(5655):223-6.
- Wallace DC. (2005) The mitochondrial genome in human adaptive radiation and disease: on the road to therapeutics and performance enhancement. Gene.354:169-80.
- Milot E, Moreau C, Gagnon A. Gagnon A, Brais B, et al. (2017) Mother’s curse neutralizes natural selection against a human genetic disease over three centuries. Nat EcolEvol. 1400–06.
- lesela S, Lukacs NW. (2021) Role of Mitochondria in Viral Infections. Life (Basel). 11(3):232.
- Choudhury AR, Singh KK. (2017) Mitochondrial determinants of cancer health disparities. Semin Cancer Biol. 47:125-46.
- Chaudhary AK, O'Malley J, Kumar S, Inigo JR, Kumar R, et al. (2017) Mitochondrial dysfunction and prostate cancer racial disparities among American men. Front Biosci (Schol Ed). 29(1):154-64.
- Xiao J, Cohen P, Stern MC, Odedina F, Carpten J, et al. (2018) Mitochondrial biology and prostate cancer ethnic disparity. Carcinogenesis.39(11):1311-19.
- Wu Y, Wang XH, Li XH, Yang SY, Peng CY, et al. Common mtDNA variations at C5178a and A249d/T6392C/G10310A decrease the risk of severe COVID-19 in a Han Chinese population from Central China. Military Med Res. 8(1):57.
- https://mail.google.clm/mail/u/0/#inbox/FMfcgzGsmrDNXRLCJWwlHNhvZBTpSFlg
- Haas RH. (2010) Autism and mitochondrial disease. Dev Disabil Res Rev.16(2):144-53.
- Uhttps://www.ncbi.nlm.nih.gov/books/NBK1422/
- Tikochinski Y, Carreras C, Tikochinski G, Vilaca ST. (2020) Population-specific signatures of intra-individual mitochondrial DNA heteroplasmy and their potential evolutionary advantages. Sci Rep. 10(1):211.
- Schurr TG, Dulik MC, Owings AC, Zhadanov SI, Gaieski JB, et al. (2012) Clan, language, and migration history has shaped genetic diversity in Haida and Tlingit populations from Southeast Alaska. Am J Phys Anthropol. 148(3):422-35.
- Wallace DC, Chalkia D. (2013) Mitochondrial DNA Genetics and the Heteroplasmy Conundrum in Evolution and Disease. Cold Spring Harbor Perspectives in Biology. 5(11):a021220.
- Barbieri C, Vicente M, Oliveira S, Bostoen K, Rocha J, et al. (2014) Migration and interaction in a contact zone: mtDNA variation among Bantu-speakers in Southern Africa. PloS one. 9(6): e99117.
- Ylikallio E, Suomalainen A. (2012) Mechanisms of mitochondrial diseases. Ann Med. 44(1):41-59.
- DiMauro S, Rustin P. (2009) A critical approach to the therapy of mitochondrial respiratory chain and oxidative phosphorylation diseases. Biochim Biophys Acta. 1792(12):1159-67.