Stem Cells as Potential Regenerative Treatment for Retinal Degenerative Diseases and Diabetic Retinopathy
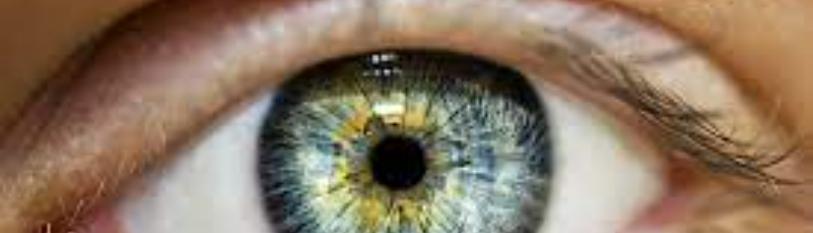
Tania Yacu and Vincent S. Gallicchio*
Department of Biological Sciences, College of Science, Clemson University, Clemson, South Carolina, USA, 29636
*Corresponding author: Vincent S. Gallicchio. Department of Biological Sciences, College of Science, Clemson University, Clemson, South Carolina, USA, 29636
Citation: Yacu T, Gallicchio VS. (2022) Stem Cells as Potential Regenerative Treatment for Retinal Degenerative Diseases and Diabetic Retinopathy. J Stem Cell Res. 4(1):1-10.
Received: December 5, 2022 | Published: January 12, 2023
Copyright© 2023 genesis pub by Yacu T, et al. CC BY NC-ND 4.0 DEED. This is an open-access article distributed under the terms of the Creative Commons Attribution-NonCommercial-No Derivatives 4.0 International License., This allows others distribute, remix, tweak, and build upon the work, even commercially, as long as they credit the authors for the original creation.
DOI: https://doi.org/10.52793/JSCR.2023.4(1)-42
Abstract
Retinal degeneration is a major cause of blindness and visual impairment in over 30 million people worldwide and can permanently destroy retinal ganglion cells. Retinal degenerative diseases result in irreversible loss of vision and can often lead to lowering one’s quality of life. Due to retinal ganglion cells being incapable of regenerating, there is a current need for finding therapeutic treatments. Mesenchymal stem cells have been identified in its therapeutic potential to reverse damage already done by these diseases and restore vision. Mesenchymal stem cells are also the most used cell type for regeneration in many pathologies. Currently, researchers have shown promising results when tested on animals, specifically rats. This review outlines the recent progress in restoring vision loss using mesenchymal stem cells. The aim of this study was to review the current use of bone marrow and adipose tissue derived mesenchymal stem cells and how researchers have applied it to retinal degenerative disease and diabetic retinopathy.
Keywords
Adipose tissue; Bone marrow; Mesenchymal stem cells; Photoreceptors; Retinal degeneration; Transplantation
Introduction
Retinal degeneration is responsible for affecting over 30 million people’s vision worldwide, which can lead to visual impairment or complete blindness [1]. Vision loss can lower one’s quality of life and length. For instance, visually impaired people are more often associated with the risk of falling, hip fractures, depression, need for social support, etc [2]. People with sensory impairments, such as vision loss, will no longer be able to participate in activities they once enjoyed, which in turn affects their mental health. Recent scientific advances have only been able to delay vision loss, but not restore it. These advances include vitamin supplements which help to delay age-related macular degeneration, intravitreal injection of a vascular endothelial growth factor inhibitor to help preserve vision, medicated eye drops and laser trabeculoplasty to reduce intraocular pressure. Early preventative treatment is also key to avoid further visual impairment. Family physicians and optometrists play an important role in acknowledging patients who are at risk and referring them to proper specialists for treatment [3].
Retinal degeneration is characterized by the loss of retinal neural cells or adjacent supporting tissue [1]. The retina is compromised with sensory tissue that encodes visual information. For instance, the neural layer of the retina works to encode this information by using photoreceptors to convert light energy into electrical signals and alter neurotransmitter release. The light energy enters the eye through the cornea and then passes through the pupil. Following the pupil the light enters the lens, which focuses the images we see before the light energy hits our retina. The two types of photoreceptors found in our retina are rods and cones. Rods are used for low light levels, whereas cones are more actively used for bright light and color vision [4]. Once light is absorbed by the photoreceptor cells, it will then be passed to the ganglion cells via the bipolar cells. These retinal ganglion cells will then form the optic nerve and send the information to the visual cortex in the brain to be interpreted into the images we see [5]. The pigmented layer of the retina or the retinal pigment epithelial (RPE) cells are responsible for absorbing light; transporting ions, nutrients, metabolites, and fluids; and secreting proteins that are necessary for photoreceptor cell survival [6].
Retinal degeneration comes in various forms, for example, one type is glaucoma which is the second leading cause of irreversible blindness, affecting over 70 million people worldwide [7]. Glaucoma is noted to cause cupping and atrophy of the optic nerve head, visual field loss, and increased intraocular pressure (IOP) [8]. Increased IOP causes damage to a mesh-like structure known as the lamina cribrosa that allows for nerve fibers to leave the optic nerve; ultimately, disrupting the normal structural and metabolic support of the retina. It is thought to be due to the increased IOP that damage to the lamina cribrosa occurs and apoptosis begins. Intraocular pressure is the fluid pressure inside the eye and uses aqueous humor production, aqueous humor outflow, and episcleral venous pressure to determine it [9]. Generally, glaucoma remains asymptomatic, and diagnosis is prolonged until it is too late for vision to be recovered. However, when diagnosis does occur, IOP reduction methods are often used but are only successful at halting or slowing down the progression and not repairing the lost vision. It is estimated that only 10-50% of people know they have glaucoma and that it mainly prevails in the older population. Symptomatic glaucoma patients often refer to their vision loss as tunnel vision and experience blurred areas that can transform to a total visual perception loss [7-9].
Another major type of retinal degeneration that causes blindness worldwide is age-related macular degeneration (AMD), with more than 20% of the aging population suffering with this disorder. With the proportion of older persons expected to grow, it has presented a need to address ways to combat incurable vision loss. Age-related macular degeneration is caused by genetic and environmental factors, such as cigarette smoking, nutritional factors, and cardiovascular diseases [10]. The two main types of AMD are neovascular (“wet”) and non-neovascular (“dry”) AMD. Non-neovascular accounts for affecting less people but has more severe outcomes regarding vision loss compared to neovascular which affects more people but is less severe. Like glaucoma, the presence of AMD in patients is often asymptomatic and causes for many to remain untreated and lead to vision loss that researchers have not found a cure to [11].
Juvenile macular degeneration are retinal degenerative diseases that will affect children and adolescents, one being Stargardt disease. Stargardt disease (STGD) is a form of macular degeneration that is most prevalent in young adults around 10-15 years of age. STGD patients often have bilateral central visual loss, meaning they have loss of vision in both eyes. The severity of Stargardt disease is dependent on the onset, the earlier the onset, the more severe the case. Patients with Stargardt disease often will have visual acuity results between 20/70 and 20/200 [12]. It affects 10 to 12.5 per 100,000 individuals in the United States and begins with central vision loss. There have been significant advances in understanding this disease both clinically and molecularly but currently, there is no treatment for the irreversible vision acuity loss that occurs [13]. Retinitis pigmentosa (RP) is another degenerative disease that affects over 2 million people worldwide and is prevalent mostly from infancy to middle age. RP is caused by the loss of photoreceptor and pigment epithelial cells, which lead to progressive deterioration of the retina and thus, progressive vision loss. Patients affected by RP often suffer night blindness and by the age of 40 will be considered legally blind [14].
Diabetic retinopathy (DR), a common ocular complication from diabetes, can also cause vision loss and blindness [15]. One third of diabetic patients are found to suffer with DR. Diabetic retinopathy is also noted to be the leading cause for vision loss in working-age populations [15,16].It is a microvascular disease that is defined by damage to the blood vessels found in the retina. Hyperglycemia, high glucose, induces vascular damage by causing the retinal blood vessels to dilate leading to loss of pericytes. Pericytes provide structural support to our capillaries. The loss of pericytes will lead to outpouching of capillary walls. Another sign of DR is apoptosis of endothelial cells and the basement membrane thickening [16]. With the prevalence of diabetes expected to increase in the coming years, it will be important to find new advances to provide a means to prevent or reverse DR.
Stem Cells
The poor regenerative ability of the retina has led to limited therapeutic processes in reversing vision loss. Some ways the retinal cells can be destroyed include light-inducing damage, oxidative stress, and inherited mutations [17]. Cell death of any of the mentioned cells can cause retinal degeneration and result in irreversible changes. Due to the lack of a cure for retinal degenerative diseases, stem cell replacement therapy has become extremely popular in providing insight in transforming the once incurable vision loss to a possible regenerative potential [18].
The idea of stem cells is that by reintroducing cells to the eye we will be able to restore vision caused by degenerative retinal diseases [18]. Stem cells can act as a repair system for the body by beginning as an undifferentiated cell and developing into a particular one [19]. While stem cells are an undifferentiated cell, they can undergo proliferation followed by cytokine stimulation to become a particular specialized cell [20]. There have been several types of stem cells used in treating retinal degenerative disease: Retinal progenitor cells (RPC), Embryonic stem cells (ESC), Induced pluripotent stem cells (iPS), Mesenchymal stem cells (MSC), and bone marrow derived VSEL. RPCs originate from fetal or neonatal retinas and generate all the retinal cells during embryonic development. Following, ESCs can become all adult cell types from the three embryonic germ layers and come from the inner cell mass of the developing blastocyst. iPSs and ESCs are similar in their developmental potential, however, iPSs originate from adult tissues. MSCs are derived from several sources, including the bone marrow, adipose tissue, placenta, cord blood and liver. These will differentiate into mesoderm type cells. Finally, VSELs are a rare type of bone marrow in mice and can differentiate into all the three germ layers. These approaches of regenerative medicine are being used to solve diseases that our body’s mechanisms have not been able to repair on its own [21]. A representation of sources of stem cells and how they can be delivered to the retina is shown in (Figure 1).
Figure 1: Representation of different types of stem cells and their arrival to the retina [20].
Discussion
Mesenchymal stem cells and their involvement with retinal degenerative diseases
Mesenchymal stem cells (MSCs) are currently the most studied type of adult stem cells for their potential involvement as a regenerative treatment for retinal diseases. These cells are most derived from adipose tissue or bone marrow and are easily isolated from these areas. They are characterized by several properties such as having a differentiation potential, ability to adhere to plastic surfaces, producing cytokines and growth factors, being involved in tissue development and regeneration, and more. MSCs ability to produce growth factors has been suggested to be a main contributor to its therapeutic potential. For retinal regeneration, MSCs can produce hepatocyte growth factor (HGF), nerve growth factor (NGF), glial cell-derived neurotrophic factor (GDNF), insulin-like growth factor-1 (IGF-1), pigment epithelium growth factor (PEGF), fibrocyte growth factor (FGF), platelet-derived growth factor (PDGF), epidermal growth factor (EGF), angiopoietin-1, erythropoietin, VEGF and TGF-β. As mentioned, MSCs can differentiate into retinal cells which has been identified by several researchers. A study was done to replicate a damaged retina combined with cultured mouse bone marrow MSCs. After the MSCs were cultured for seven days, results showed that MSCs differentiated to having retinal cell markers and producing neurotrophic factors [21].
Current research of mesenchymal stem cells and its involvement with retinal degenerative diseases and diabetic retinopathy
A study published in 2017 by Ben Mead and Stanislav Tomarev, was done to investigate for the first time if bone marrow mesenchymal stem cells-derived exosomes provided any neuroprotective and axon regeneration potential on injured retinal ganglion cells. As retinal ganglion cells are the leading cause of retinal degenerative diseases. The study was performed by purchasing two groups of cultures: human BMSC (variable group) and human fibroblasts (control group) and isolating their exosomes. 36 adult rats were used in this experimental design and split into 6 groups based on their injury and treatment plan. The exosomes were then delivered to Groups 3-6 via a 33g Hamilton syringe injected into their vitreous humor, as shown in (Figure 2) [23].
Figure 2: Representation of the experimental design of the study in a timeline manner [23].
Electroretinography (ERG) records were performed on day 0 and 21 to test the electrical response of the eye’s intensity to a known light. Optical Coherence Tomography (OCT) was also used to examine the rat’s eye by taking images of the retina near the optic nerve to allow the researchers to detect segmentation of the retinal nerve fiber layer and calculate the thickness. On day 21, the rats were sacrificed, and their eyes and optic nerves were removed to be prepared as a tissue slide. These retinal cell slides then underwent immunocytochemistry and immunohistochemistry. Researchers used the optic nerve crush (ONC) model of the CNS injury [23]. The ONC model was developed to allow for researchers to analyze retinal ganglion cell survival. It is a helpful model when researchers want to see if a substance will have any effects on RGC death and survival [24]. In the case of the experiment performed by Ben Mead and Stanislave Tomarev, the ONC model showed that BMSC-derived exosomes had significant neuroprotective and axon regeneration effects on maintaining retina function. Compared to untreated retina, researchers saw 80-90% retinal ganglion cell loss, where BMSC-derived exosomes only were shown to have 30% loss. This is an important finding because retinal ganglion cells are CNS neurons, making them not regeneratable. The OCT and ERG data were observed to compare retinal ganglion cells' axon protection and function in the untreated, fibroblast exosome, and BMSC exosome treated retina. Results showed that BMSC-derived exosomes offered 50% of RGC function to be maintained and protected, whereas the untreated and fibroblast exosomes offered no protection or preservation of RGC function [23].
Another experiment published in 2006 by Arnold and team, researched the effects of transplanting bone marrow-derived mesenchymal stem cells in the affected retina of rhodopsin knockout mice to see if photoreceptor cells could be saved. The purpose of this experiment was done to understand the potency of mesenchymal stem cells in rescuing effects caused by retinal degenerative diseases, specifically retinitis pigmentosa. Retinitis pigmentosa is characterized by its loss of retinal photoreceptor cells that also cause the loss of peripheral vision in patients. The experiment involved 3 experimental groups (Group One: untreated rhodopsin knockout (control group), Group Two: rhodopsin knockout mice injected with mesenchymal stem cells, and Group Three: rhodopsin knockout injected only with medium) containing 10 rhodopsin knockout mice each [25].
All the mice were anesthetized with Ketanest/Rompun to allow for researchers to inject their right eyes, left eyes remained untreated. In group two, the mice were injected with 60,000 vector-transduced mesenchymal stem cells and Group three received the same amount but using Hank’s balanced salt solution instead. Retinas were then examined using a Leitz stereomicroscope in the two experimental groups to see if any mice had massive subretinal hemorrhage, vitreous hemorrhage, or large retinal detachments. If so, these mice would be disregarded from the study. For researchers to investigate if cytoplasmic markers would appear, the mice underwent staining procedures. To study and evaluate the rescue effects of this experiment, researchers removed the corneas of the eyes and processed them to allow for light and electron microscopy to take place. The researchers quantified rescued effects with the Axiophot light microscope [25].
The results of this experiment were obtained on the 35th day of the MSCs injection. The rescue effects were assessed in the semi-thin sections of the retinas of the three groups by looking for the presence of several photoreceptor nuclei shown in (Figure 3). In Groups One and Three, there were hardly any rows of preserved photoreceptors (0.4±0.3 rows in the control group and 0.9±0.3 in the rhodopsin knockout injected only with medium) compared to in the MSCs injected group that had 2.25±0.4 rows of preserved photoreceptors [25]. The findings of this study support the idea that implementing mesenchymal stem cells to retinal injuries/defects could stimulate rescue effects.
Figure 3: Representation of the semi-thin sections of rho-/- retinas and their rescue effects in a) untreated control b) MSCs injected group and c) rhodopsin knockout injected only with medium group [25].
Research done by Yuji Inoue and his team, summarize that using mesenchymal stem cells can delay photoreceptor cell apoptosis, meaning that MSC secreted factors could be a useful cell source for photoreceptor cell survival. The goal of this study was to understand if stem cell therapy can be replaced for defective retinal cells. Researchers isolated mesenchymal stem cells from Pcrx2K-lacZ transgenic mice and inserted them into the anterior chamber of rats. The rats were then sacrificed for their eyes to be removed and studied. As shown in (Figure 4), when comparing total cell number between the conditioned medium and the control culture, there was a 1.6-fold higher number of total cells and 1.4-fold higher increase in the number of photoreceptor cells found in the cells treated with the conditioned medium. Using electroretinograms waveforms, it was revealed that rats with MSC transplantation were delayed in having retinal degeneration. In conclusion, this study shows potential in how MSCs can slow down photoreceptor loss [26].
Figure 4: Representation of how the conditioned medium from bone marrow mesenchymal stem cells can promote photoreceptor cell survival. (A) represents the retinal cell cultures in the control versus conditioned treatment. (B) portrays total cell number (left panel) and photoreceptor cell viability using β-gal assay (right panel) of the control versus conditioned treatment [26].
In 2020 Kahraman,et. al., performed a study following up on eight patients with degenerative macular diseases who had adipose tissue derived mesenchymal stem cells implanted in one of their eyes. These patients either were clinically diagnosed with age-related macular degeneration or Stargardt’s macular dystrophy. The researchers identified their use for adipose tissue instead of bone marrow derived MSCs, because they were able to harvest it easier, have a faster expansion, and higher protein secretion. The goal was to evaluate retinal diseases where curative treatment is not available and see if stem cell therapy had any potential. The results of the study included those seven out of the eight patients had improvements in their visual acuity and visual field exams, with their fellow eyes worsening. The results of this study provide evidence of how adipose-derived stem cells can be beneficial in treating future retinal degenerative diseases [27].
A meta-analysis was conducted by Gaddam,et. al., in 2019 to investigate how mesenchymal stem cells can help to reverse lost vision caused by diabetic retinopathy by looking at different clinical trials. One study found that both adipose stem cells and bone marrow-derived mesenchymal stem cells were promising options for recovering the retina. This is partly since adipose stem cells have been found to be functionally and phenotypically like pericytes. The study was performed on rats who presented with the early-stage symptoms of diabetic retinopathy. The rats were injected with adipose stem cells in their eyes and monitored for any retinal function improvement. Using an electroretinogram, the results found that the rats had a significant decrease of leakage and apoptotic cells near the blood vessels. This means that the adipose stem cells injected appear to improve the retinal function for these rats. The adipose stem cells were also shown to differentiate into pericytes, which is often lost in patients with diabetic retinopathy. Another study administered adipose stem cells into the vitreous cavity of diabetic mice’s eyes. This study found that the adipose stem cells can reduce oxidative damage and prevent RGC loss from occurring. This is an important finding because RGC loss is a key event for the onset of diabetic retinopathy. Currently, there are multiple human clinical trials being performed on the use of adipose and bone marrow mesenchymal stem cells and its effects on repairing retinal damage. An example of one of these clinical trials include bone marrow mesenchymal stem cells being given to subjects with irreversible vision loss caused by diabetic retinopathy. However, these trials are still in their pilot phases and are awaiting approval for their safety and feasibility of conducting them [28].
Ethics and Controversy
General use of stem cells as a potential treatment
Before mesenchymal stem cells are constituted as a feasible approach for treating retinal degeneration, many issues will need to be resolved. The first issue being concerned with safety of retinal stem cell transplantation. Many experts worry that because of public awareness towards the potential success of stem cell therapies, that many industries will commercialize these interventions too soon or without proper design of clinical studies. Resulting in a raise of safety concerns. Another safety aspect to be considered is involving the transplantation techniques during surgery. The current surgical techniques in delivering stem cells have risk factors that must be addressed, such as retinal perforations, detachment, tissue damage, etc., which may result in more damage to a patient’s vision [1]. Other risk factors of stem-cell based interventions involved tumors forming, patients having an immunological reaction, cells not behaving properly, and long-term health effects. This is since we have limited experience of how mesenchymal stem cells work in humans, which can cause adverse effects or failure of improvement at all in patients [29].
Stem cell research has also posed ethical and political controversies, especially dealing with informed consent. Many times, people in the phase I clinical trials expect that they will benefit from the trial, however, that is not the case. Phase I trials are meant to study the safety of the experiment and not the efficacy. This leads to a “therapeutic misconception” and many patients overestimate their benefits. It will be imperative for researchers to educate patients on the role of the clinical trial and not to give unrealistic hopes to them [29]. Ethical issues like this will need to be discussed to provide appropriate stem cell research in the future.
Future Recommendations
Future studies should focus on having larger sample sizes when researching. For instance, this will help to optimize that the results are more closely generalized to the population and give more reliable results. To prevent a decrease in statistical power and an increase in margin of error, it will be important to increase sample size. Additionally, current clinical trials are mostly only being conducted on animal models. A future recommendation would be to conduct these same trials on humans in a safe and ethical manner to help confirm the therapeutic potential of stem cell treatment on retinal degenerative diseases. Evidence of safety should also be established before performing each trial.
Lastly, future participants should have realistic understandings of clinical trials and understand the risks that may occur. Further work should also be done to provide consistency of the results in previous studies. It will be important to gather more data to understand thoroughly the risk-to-benefits for each of these clinical trials. With continued research and development of retinal degeneration and mesenchymal stem cells, there shows promising potential in treatment.
Conclusion
Although there are still many issues to be addressed before approving mesenchymal stem cell therapy as a curative treatment, the results obtained from the studies above offer a promising option for the future. This prospective option of using adipose-derived or bone marrow-derived mesenchymal stem cells will influence improving the quality of life of many people suffering from retinal degenerative diseases and diabetic retinopathy. With continued efforts in developing and researching stem cell biology, there is hope in treating the current “irreversible” retinal degenerative diseases and diabetic retinopathy that many are and will be affected with.
References
1. Singh MS, Park SS, Albini TA, Canto-Soler MV, Klassen H, et. al. (2020) Retinal stem cell transplantation: Balancing safety and potential, Prog Retin Eye Res. 75:100779.
2. Misajon R, Hawthorne G, Richardson J, barton J, Peacock S, et. al. (2005) Vision and quality of life: the development of a utility measure. Invest Ophthalmol Vis Sci. 46(11):4007-15.
3. Allen P, Ledy R, Coffin J. (2016) Vision Loss in Older Adults. Am Fam Physician. 94(3):219-26.
4. Hoon M, Okawa H, Della Santina L, Wong RO. (2014) Functional architecture of the retina: development and disease. Prog Retin Eye Res. 42:44-84.
5. Lev S. (2001) Molecular aspects of retinal degenerative diseases. Cell Mol Neurobiol. 21(6):575-89.
6. Simó R, Villarroel M, Corraliza L, Hernandez C, Garcia-Ramirez M, et. al. (2010) The retinal pigment epithelium: something more than a constituent of the blood-retinal barrier--implications for the pathogenesis of diabetic retinopathy. J Biomed Biotechnol. 2010:190724.
7. Weinreb RN, Aung T, Medeiros FA. (2014) The pathophysiology and treatment of glaucoma: a review. JAMA. 311(18):1901-11.
8. Thylefors B, Négrel AD. (1994) The global impact of glaucoma. Bull World Health Organ. 72(3):323-6.
9. Kang JM, Tanna AP. (2021) Glaucoma. Med. Clin. N. 105(3):493-510.
10. Lim LS, Mitchell P, Seddon JM, et. al., Age-related macular degeneration. Lancet. 2012; 379(9827):1728-38.
11. Thomas CJ, Mirza RG, Gill MK. Age-Related Macular Degeneration. (2021)
Med. Clin. N. 105(3): 473-91.
12. Tanna P, Strauss RW, Fujinami K, Michaelides M. (2017) Stargardt disease: clinical features, molecular genetics, animal models and therapeutic options. Br J Ophthalmol. 101(1):25-30.
13. Lu LJ, Liu J, Adelman RA. (2017) Novel therapeutics for Stargardt disease. Graefes Arch Clin Exp Ophthalmol. 255(6):1057-1062.
14. Jin ZB, Gao ML, Deng WL, Wu KC, Sugita S, et. al. (2019) Stemming retinal regeneration with pluripotent stem cells. Prog Retin Eye Res. 69:38-56.
15. Ning CP, Mitchell, et. al., Diabetic retinopathy. The Lancet. 2010. 376(9735):124-36
16. Wei W, Amy L. (2018) Diabetic Retinopathy: Pathophysiology and Treatments. National Library of Medicine. 19(6):1816.
17. Athanasiou D, Aguilà M, Bevilacqua D, Novoselov SS, Parfitt DA, et. al. (2013) The cell stress machineryand retinal degeneration. FEBS Lett. 587(13):2008-17.
18. Jeon S, Oh IH. (2015) Regeneration of the retina: toward stem cell therapy for degenerative retinal diseases. BMB Rep. 48(4):193-9.
19. McCulloch EA, Till JE. (2005) Perspectives on the properties of stem cells. Nat Med. 11(10):1026-8.
20. Rao RC, Dedania VS, Johnson MW. (2017) Stem Cells for Retinal Disease: A Perspective on the Promise and Perils. Am J Ophthalmol. 179:32-38.
21. Huang Y, Enzmann V, Ildstad ST. (2011) Stem cell-based therapeutic applications in retinal degenerative diseases. Stem Cell Rev Rep. 7(2):434-45.
22. Holan V, Palacka K, Hermankova B. (2021) Mesenchymal Stem Cell-Based Therapy for Retinal Degenerative Diseases: Experimental Models and Clinical Trials. Cells. 10(3):588.
23. Mead B, Tomarev S. (2017) Bone Marrow-Derived Mesenchymal Stem Cells-Derived Exosomes Promote Survival of Retinal Ganglion Cells Through miRNA-Dependent Mechanisms. Stem Cells Transl Med. 6(4):1273-85.
24. Tang Z, Zhang S, Lee C, et. al., An optic nerve crush injury murine model to study retinal ganglion cell survival. J Vis Exp. 2011; (50):2685.
25. Arnhold S, Absenger Y, Klein H, et. al.,Transplantation of bone marrow-derived mesenchymal stem cells rescue photoreceptor cells in the dystrophic retina of the rhodopsin knockout mouse. Graefes Arch Clin Exp Ophthalmol. 2007; 245(3):414-22.
26. Inoue Y, Iriyama A, Ueno Sh, Takahashi H, Kondo M, et. al. (2007) Subretinal transplantation of bone marrow mesenchymal stem cells delaysretinal degeneration in the RCS rat model of retinal degeneration. Exp Eye Res. 85(2):234-41.
27. Kahraman NS, Gone ZB, Sevim DG, Oner A. (2021) First Year Results of Suprachoroidal Adipose Tissue Derived Mesenchymal Stem Cell Implantation in Degenerative Macular Diseases.Int j Stem Cells. 14(1): 47-57.
28. Sriprachodaya G,Periasamy R, Gangaraju. (2019) Adult Stem Cell Therapeutics in Diabetic Retinotherapy. Int J Mol Sci. 30;20(19):4876.
29. Lo B, Parham L. (2009) Ethical Issues in Stem Cell Research. Endocr Rev. 30(3): 204-13.