Regional Variations and Interrelationships of Age and Polyparasitism on Covid-19 in Africa
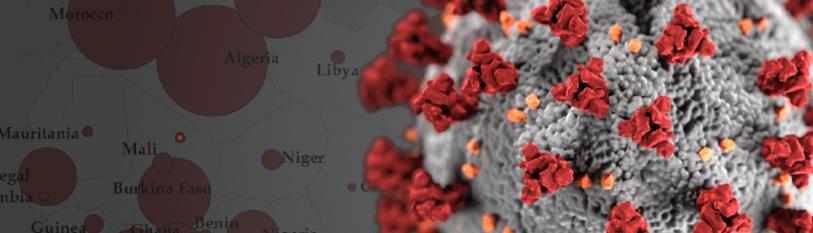
Fatimah L.C Jackson1*, Raven Flowers2, Nicholas Guthrie3, Hasan Jackson4, Kaitlin Keaton1,2, Jade Telesford1,2 and Obinna Asawabelem1,2
1,2Howard University and Quad Grid Data Lab
3George Washington University and Quad Grid Data Lab
4 QuadGrid Data Lab
*Correspondingauthor: Fatimah LC Jackson, Howard University and Quad Grid Data Lab
Citation: Jackson FLC, Flowers R, Guthrie N, Jackson H, Keaton K, et al. (2023) Regional variations and Interrelationships of age and polyparasitism on COVID-19 in Africa. Adv Clin Med Res. 4(3):1-20.
Received: August 17, 2023 | Published: September 11, 2023
Copyright© 2023 genesis pub by Jackson FLC, et al. CC BY-NC-ND 4.0 DEED. This is an open-access article distributed under the terms of the Creative Commons Attribution-Non Commercial-No Derivatives 4.0 International License., This allows others distribute, remix, tweak, and build upon the work, even commercially, as long as they credit the authors for the original creation.
DOI: https://doi.org/10.52793/ACMR.2023.4(3)-62
Abstract
The patterns of COVID-19 infection in Africa remain something of an enigma. The continent has very high rates of existing parasitemia, particularly in the regions south of the Sahara Desert, has grossly underfunded health care resources, limited access to COVID-19 vaccines, yet appears to have contained the intensity of the COVID-19 pandemic. No specific human genetic adaptations to COVID-19 are expected, given the recency and non-African origins of the specific responsible virus. Broadly distributed existing human genetic polymorphisms may secondarily affect COVID-19 attachment abilities and present the virus with a diverse host environment. The young age of the African population and high levels of existing immunostimulatory polyparasitism, particularly due to intestinal helminths, may stand in the way of explosive continent-wide COVID-19. We propose a coupled model of proximal causes, age and polyparasitism influencing COVID-19 susceptibilities. Regional variation exists within Africa in COVID-19 intensity, suggesting that additional local biocultural factors may be contributing to COVID-19 infection at the local level.
Keywords
Helminthiasis; Youth; SARS-CoV-2; COVID-19 diathesis; Adaptive interventions in infectious disease
Introduction
In Africa, communicable and infectious diseases predominate in stark contrast to the rest of the world where non-communicable disease predominates [1]. Additionally, many infectious diseases have emerged or reemerged in Africa in the 21st century [2]. Broad swaths of tropical Africa maintain high exposure and susceptibility to such viruses as Ebola, Zika, and Chichunguka [3].
Given that Africa is the most tropical of continents and the homeland of our species, Homo sapiens, it is logical to surmise that extended exposure to tropical African biomes and the ubiquity of communicable and infectious diseases in these regions would suggest that infectious diseases have modified the genomes of our ancestral as well as contemporary populations. Protracted endemic exposures to communicable and infectious disease has been the norm for most indigenous populations in Africa for innumerable generations [4]. Because of the centuries of endemic infectious disease exposures, it is not clear now how specific aspects of African human genetic diversity, related to infectious diseases differs among indigenous Africans, although it is apparent that ancestry has a direct impact on an individual’s immune response to infectious diseases [5]. While it is true that host genetic factors, along with other risk factors may help determine susceptibility to respiratory tract infections, the genetic risk factors for SARS-CoV-2 which explain differences within the African context are not yet known [6]. The relationships of African genetic representation in the context of SARS-CoV-2 infection and COVID-19 severity were the recent subject of a review paper from South Africa [6]. The researchers detailed the many forces (economic, educational, political, etc.) that have mediated against meaningful intracontinental comparisons. Our research confirms these findings of limited intracontinental comparisons, particularly on the topic of genetic diversity in response to SARS-CoV-2. As biological anthropologists and evolutionary biologists, we are naturally drawn to understanding how human genetic variation impacts COVID-19 susceptibilities, but our database is currently limited. SARS-CoV-2 invaded an African landscape already beset by centuries of exposure to hyper immunostimulatory infectious diseases. This meant that to develop into COVID-19 the coronavirus had to contend with the existing high levels of African genetic diversity and already amplified immunocompetence.
Despite this, one of the earliest hypotheses of human genetic diversity’s role in response to COVID suggested the role of a polymorphism in the angiotensin-converting enzyme 2 (ACE2) gene. ACE 2 that has been shown as a genetic risk factor for SARS-CoV-2 infection and is required by the virus to enter cells [7]. The most common ACE2 polymorphisms in Africans are (in order of descending population saturation) rs142017134 (100%), rs147311723 (80%), rs464179 (60%), and rs147404721 (25%). These are considered destabilizing SNPs in SARS-CoV2-ACE2 protein and spike glycoprotein and likely have adverse implications for virus entry mechanisms [8]. Of particular importance is rs142017934, which is expressed at near fixation levels among Africans, amplifying the expression of the zinc metalloproteinase ACE2 gene [51]. Changes in ACE2 genetics influences expression and folding structure, leading to changes in function and miRNA binding, which could influence COVID-19 susceptibility. Yet, the ubiquity of this and other ACE2 polymorphisms in contemporary Africans suggests that they have been under other, prior positive selective forces since ACE2 is highly expressed in cardiovascular and kidney tissues and involved in the renin-angiotensin system [9].
Additional hypotheses on human genetic diversity implicate transmembrane protease serine 2 (TMPRSS2) and dipeptidyl peptidase-4 (DPP4), which may also play important roles in disease severity [8]. SARS-CoV-2, for example, is spread by airway contact and uses TMPRSS2 to facilitate viral entry and spread. Host-expressed TMPRSS2 on nasal and bronchial epithelium activates the SARS-CoV-2 spike protein and cleaves the ACE-2 receptor to which the virus binds, enabling SARS-CoV-2 to enter the body [10]. The linear regression of TMPRSS2 expression was found to be significantly higher in individuals of recent African descent compared with non-Africans [11]. Dipeptidyl peptidase 4 (DPP4; also known as CD26) is a functional receptor for human coronavirus [12]. In bats, DPP4 genes have been subject to significant adaptive evolution and at least three DPP4 genetic variants appear to interfere with the viral surface glycoprotein. In humans, DPP4 is a receptor for Middle East respiratory syndrome coronavirus (MERS-CoV), a disorder that shares 80% similarity with COVID-19 [9]. Unlike TMPRSS2, DPP4 is not concerned with the entry of COVID-19 but mediates the inflammatory reactions and cytokine storm that induced by COVID-19 [9].
Therefore, we are limited in knowing the exact relationship between existing African human genetic variability and its regional relationship with infectious disease risk for SARS-CoV-2 for three main reasons. First, comprehensive assessments of African human genetic diversity are still under construction, including a comparative African Human Genomic Reference Database [13]. Second, many of the most recent infectious disease studies are on African migrants to Europe and North America [14]. However, since these migrants do not necessarily represent a representative cross-section of indigenous African populations, it is not clear that the patterns observed among African migrants reflect the importance of these diseases on the African continent. Thirdly, unlike earlier coronaviruses, SARS-CoV-2 is a recent pathogenic invader to Africa for which regional distinctions may not have yet saliently emerged.
Our general knowledge about the interrelationship of African human genetic diversity and infectious diseases comes from the many studies of malaria and genetic adaptations to malaria. Often, mutations in response to longstanding infectious diseases have high selective values and become quickly disseminated across geospatial regions and among diverse African ethnic groups. Excellent examples of this include the APOL1 polymorphisms associated with resistance to Human African Trypanosomiasis (sleeping sickness) [15], the Duffy blood group polymorphism associated with resistance to P. vivax malaria [16], and the distribution of the sickle cell mutation and variants in glucose-6-phosphate dehydrogenase in response to endemic P. falciparum malaria [17].
COVID-19 caused by the coronavirus SARS-CoV-2 is just the latest wave of pathogenic viruses to sweep into Africa. What is unusual is that, in contrast to elsewhere in the world, the virus is not resulting in as many infections and deaths. It may be that the cadence of transmission and the magnitude of morbidity and mortality may have taken on unique African characteristics, given the existing template of endemic infectious and communicable diseases [18]. So far, the ongoing pandemic has mainly severely damaged the world's most economically developed countries and remains a major threat for low- and middle-income countries [19]. As Africa is currently the least economically developed continent with the weakest health infrastructure [20] early in the pandemic it was predicted that Africa would be significantly adversely affected by the COVID-19 outbreak [21]. As we approach 2024, this dire prediction has fortunately not yet been fulfilled.
Twenty years ago, the Institute of Medicine identified the key factors influencing microbial disease dynamics worldwide, with most of these factors being over-represented in Africa [22]. The IOM’s list included microbial adaptation and change, human susceptibility to infection, climate and weather, changing ecosystems, human demographics and behavior, economic development and land use, international travel and commerce, technology and industry, breakdown of public health measures, poverty and social inequality, war and famine, lack of political will and intent to harm [22]. In this paper, we examine some of the most relevant Africa-specific intervening factors suspected to influence the epidemiology and disease diathesis of COVID-19 on the continent. We propose a coupled model of proximate contributors (age and polyparasitemia) that together explain the overall lower rate of SARS-CoV-2 infection across the African continent, we identify the likely physiological underpinnings of these interrelationships, and then we place these findings within an evolutionary context.
Methods and Materials
To comprehensively evaluate COVID-19 in Africa and place our findings in an evolutionary context, we consulted the latest information on the distribution, intensity, and duration of the SARS-CoV-2 virus throughout the continent on a country-by-country basis. Three electronic databases (i.e., PubMed, EMBASE, and ISI Web of Science) were searched without language restrictions. Relevant data were extracted and random-effects meta-analyses conducted.
The Ensembl genome browser v. 107 (July 2022) (https://useast.ensembl.org/index.html) was consulted for information on genomic variation in ACE2. The Genome Aggregation Database (gnomAD browser) (https://gnomad.broadinstitute.org/) was used to investigate structural and functional changes in ACE2 due to polymorphism.
Peak infections in a pandemic curve, as determined by Infectious disease and statistical modeling specialists, implies that the number of new cases has begun to level off rather than continuing a sharp upward trajectory. Pandemic curves are usually symmetrical in nature with a sharp increase of cases is followed by the plateau or peak, which then gives way to a decline in new diagnoses. Using data from the public access Country Profiles Database of Our World in Data’s Coronavirus Pandemic (COVID-19) Database we tracked the reported incidence of COVID-19 morbidity and mortality on a country-by-country basis to calculate peak infections. Each country profile included the number of confirmed daily new cases, the number of confirmed cases since the pandemic began, any changes in the case loads, the number of deaths reported, the extent of vaccination doses administered every day and the proportion of the population to receive vaccination, the extent of COVID-19 testing being done to uncover COVID-19 cases, and the general government response to the pandemic.
African countries and regions were defined using ISO definitions (ISO 3166) of United Nations designated entries and the online browsing platform. Maps were constructed using ArcGIS, Regional clusters were determined following United Nations designations [23] and regional anomalies (in COVID-19 prevalence) were identified when the peak infection levels differed significantly within or between ecologically and ethnographically contiguous areas of the continent. Comparisons in COVID-19 were made within and between geoschematic regions of Africa.
All data used in this paper are publicly available through the World Health Organization and CDC-Africa. Data in tables were calculated by the authors based upon publicly available data sites.
In this study, limitations in the quantity and quality of country-specific data must be noted. Some countries counted their COVID-19 morbidities and mortalities differently and this altered some of our totals. Also, other African countries didn’t count daily but rather weekly totals. In constructing our totals (see Figures 1 and 2 below), we made comparisons over time across and between regions after standardizing the available data.
Figure 1: Progression of confirmed COVID-19 cases from January 2020 to June 2022 on the African continent.
Figure 2: Modified United Nations geoscheme for Africa. COVID-19 morbidity and mortality rates within and between each of these official regions are compared to detect regional anomalies in the prevalence of COVID-19.
Results and Discussion
Worldwide variation in covid-19 morbidity and mortality
Compared with other parts of the world, the incidence, intensity, and mortality due to SARS-CoV-2 is much less in Africa than elsewhere (see Table 1), consistent with previous studies [18][6]. This is something of an enigma, however. Throughout the world, the SARS-CoV-2 virus is influenced by local dynamics. We have identified 10 major factors, enumerated below, that may differentially influence the continent-wide expression of SARS-CoV-2. These 10 factors are taken into consideration in developing our proximate coupled model of COVID-19 in Africa.
Location |
Total Population1,2 |
CIVID 19 Cases |
COVID-19 cases per 1M resident |
COVID-19 deaths per 1M resident |
Total Covis-19 deaths |
Worldwide |
7,960, 000,000 |
572, 396, 969 |
73,612 |
80.3 |
63,88,967 |
United States |
32,95,00,000 |
9,05,89,023 |
2,74,851 |
311 |
10,24,372 |
India |
1,38,96,37,446 |
4,30,39,764 |
32,295 |
379 |
526, 167 |
Brazil |
21,72,40,060 |
3,36,59,879 |
1,59,271 |
312 |
6,77,494 |
France |
6,46,26,628 |
3,27,23,412 |
4,87,856 |
229 |
1,48,306 |
Germany |
8,37,83,942 |
3,05,98,385 |
3,67,993 |
171 |
1,43,545 |
United Kingdom |
6,78,86,011 |
2,32,12,565 |
3,49,400 |
269 |
1,82,727 |
Italy |
6,04,61,826 |
2,07,72,833 |
3,44,815 |
284 |
1,71,232 |
South Korea |
5,12,69,185 |
1,94,46,946 |
3,75,564 |
49 |
24,932 |
Table 1: Non-African countries with highest number of COVID-19 cases.
- Corona viruses have zoonotic potential due to the adaptability of their S protein to receptors of other species, most notably demonstrated by SARS-CoV-2, the causative agent of the SARS epidemic, which probably originated from bats [23-24]. The availability of and access to zoonotic hosts is highly influenced by local environmental conditions.
- The genomic sequence of SARS-CoV-2 indicates it belongs to the betacoronavirus genus, an identity of 80–96% is reported with SARS-CoV-2 and 50% with MERS-CoV that originate in bats (and some of the other zoonotic species implicatedin SARS-CoV2 transmission)[25-27]. The presence of bats is non-uniform across diverse geographical ranges and the density of bats is correlated with a number of ecological and societal variables.
- Of the diverse subtypes of this virus, betacoronaviruses can cause severe illness and death, while alphacoronaviruses cause mild or asymptomatic infection. This suggests inherent variations in the subtypes as they interact with the environment: gene-environment interactions dictate the magnitude of pathology. The mean incubation period was 6.38 days 95% CI (5.79; 6.97); range 2.33–17.60 days [28]. The incubation period of the SARS-CoV-2 B1.1.7 variant, for example, is shorter than that of other strains [29].
- This virus has the ability, due to its structure, to produce the receptor binding of ACE2 from host cells [30-34]. Since human populations differ in the distribution of specific variants of ACE2 receptors, this factor is thought to influence the proliferation of the virus.
- The incubation period and the onset of symptoms of SARS-CoV-2 is an average of 5.2 days, it is reported that the death of the infected person occurs between this period of 6 and 41 days with an average of 14 days. Variation in the incubation period and onset of symptoms suggests environmental modulation.
- The main reported symptoms of the upper and lower respiratory tract are dry cough, runny nose, sore throat and dyspnea, associated with headache and fever. Some patients have reported gastrointestinal symptoms such as diarrhea or even have very mild symptoms or can be asymptomatic carriers. Bilateral opacities in the form of ground glass are identified on chest radiographs and tomography [35][27]. Given this, some researchers suggested that we analyze urine and fecal samples to exclude or identify alternative routes of transmission that can influence viral dynamics.
- Evidence suggests that person-to-person transmission is the likely route to spread COVID-19 infection [36-40][32-34]. Person-to-person transmission is highly influenced by non-genetic factors, such as cultural practices within communities (e.g., traditional social distancing, gender segregation, etc.).
- Transmission is also facilitated by contact with contaminated surfaces through drops that expand when coughed or sneezed [41-44]. The presence of contaminated surfaces and community access to these are another set of environmental factors influencing viral transmission patterns.
- The main cases of corona virus death occur mainly in older people, probably due to their poorer immune systems. Thus, age has an impact on viral dynamics through the lessening of immune competence with age. An example of this in the Western World was the well vaccinated 84-year-oldretired US General Colin Powell suffered from multiple myeloma and died from COVID-19 after becoming infected while his immune system was severely compromised by cancer.
- Adults and children with underlying noncommunicable diseases such as diabetes, chronic cardiovascular and lung diseases or even high blood pressure and cancer are at a higher risk of coronavirus infection [45-46] [42-43] [47-49]. This reminds us that pre-existing conditions can modify the settings within which SARS-CoV-2 can thrive and persist.
Covid-19 in the African Context and Worldwide
As Figure 1 shows, Africa is characterized by localized cases of COVID-19, usually concentrated in the densely-populated capitol regions. This is in contrast to the broader urban and rural distribution of the COVID-19 pandemic outside of Africa. Figure 3 depicts the distribution and transition of confirmed cases of COVID-19 within Africa from January 2020 to June 2022.
The African pattern is in distinction to the pattern of COVID-19 elsewhere in the world was presented in Table 1.
Worldwide, there are currently a total of 572,396,969 SARS-CoV-2 cases. As of July 25, 2022, deaths reached 6,388,967 worldwide. Table 2 presents the countries with the top number of cases of confirmed COVID-19, deaths due to COVID-19 per million residents, total deaths, and mortality rate from morbidity per million residents.
Location |
Total Population 1,2 |
Total COVID-19 morbidity 1,2 |
COVIS-19 cases per 1M residents |
COVIS-19 death per 1M residents |
Total COVID-19 morality |
African Continent |
1,40,67,64,498 |
24,23,000 |
190 |
18 |
2,56,000 |
Worldwide |
7,96,00,00,000 |
572.396.969 |
73,612 |
80.3 |
63,88,967 |
Table 2: Comparison of COVID-19 morbidity and mortality in Africa versus the World.
Human Genetic Variability and COVID-19 Susceptibility
SARS-CoV-2 susceptibility and COVID-19 disease severity are associated with genetic variants affecting gene expression in a variety of tissues. Genetic variability in the host populations is a strong indicator that positive selection could affect the ability of a virus to proliferate within that population. The continent of Africa continues to maintain the greatest degree of human genetic variability worldwide. However, given the recency of SARS-CoV-2 in Africa, it is unlikely that any relevant human genetic variability differs across the African continent or significantly interacts with this new coronavirus. SARS-CoV-2, emerging from Wuhan, China in December 2019 and then spreading worldwide [8], was introduced into Africa in 2020 and is thus exogenous to the continent’s indigenous people. Therefore, the African people’s contact with this virus is of recent duration. Even for the viruses with deeper African roots such as HIV and Ebola, we do not see significant intracontinental regional differences in susceptibility. Soon after HIV-AIDS was recognized, for example, researchers in the DRC cloned and sequenced the complete genomes of two isolates obtained from Congolese patients. Their genetic organization is identical with that of isolates from Europe and North America, confirming a common evolutionary origin. However, the comparison of homologous proteins from these different isolates differed and this may be the level at which human genetic variability in Africa may interface with SARS-CoV-2 disease expression.
Africa’s Demographic Age Structure and COVID-19 Epidemiology
In Africa 2050, the Hoover Institute reports a profound but simple fact about the African continent: No general statement about African demography is true. The variation in the continent is too great for any single generalization. Africa today includes giant countries with populations near or exceeding 100 million (Egypt, Ethiopia, Nigeria) and tiny countries with populations under 1 million (Comoros, Djibouti, Cabo Verde, Reunion, Mayotte, Sao Tome and Principe, Seychelles). It includes countries where fertility is rising (Algeria, Egypt, Tunisia, Seychelles), countries where fertility is high but stable, falling by less than 1% per year (Mozambique, the Democratic Republic of the Congo, Nigeria, and ten others), and countries where fertility is high but falling very rapidly, 2.5% per year or more (Ethiopia, Rwanda, Kenya, Malawi, and Sierra Leone). It also includes countries where fertility rates are exceptionally high, exceeding six children per woman (Niger, Somalia, Chad, DRC, Mali) and countries where fertility has fallen to replacement levels (2.1) or below (Tunisia, Mauritius). Annual population growth rates for African countries range from under 0.5% per year (Mauritius, Central African Republic, Libya) to eight times that rate, or about 4% per year (Niger, Equatorial Guinea). Some African countries are highly urbanized, with urban populations around 60% of their total (South Africa, Angola, Botswana), while others are barely urbanized at all, with urban populations less than 20% (Burundi, Ethiopia, Malawi, Niger, Rwanda, Uganda). And while Africa has most of the world’s highly ethnically diverse countries, some African countries are quite low in ethnic diversity (Burundi, Algeria, Tunisia, Egypt).
The indigenous peoples of Africa are a growing segment of the world community and these populations tend to be younger. The large proportion of African youth reflects the historical pro-natal cultural priorities of East, West, and Central Africans coupled with the disconnect between female education and fertility in these regions. In 1950 the population of Africa was 177 million and it grew 7.6 times to more than 1.341 billion in 2020. Now, Africa is the continent with the youngest population worldwide. As of 2021, around 40 percent of the population is aged 15 years and younger, compared to a global average of 26 percent [50]. Fertility is also highly variable within Africa; South Sudan and Angola have some of the highest population growth rates, totaling 3.83 percent and 3.52 percent, respectively [50]. Africa is quickly recovering from the destructive population losses associated with centuries of extractive enslavement by Europeans, Arabs, and other Africans, massive population displacements facilitated by wars, exploitative colonialization by European and Arab powers, years of local political mismanagement precipitated by low educational levels, and ongoing virulent endemic infectious and highly communicable diseases. What this means is that the demographic structure of Africa is younger than populations elsewhere and since vulnerability to SARS-CoV-2 is in part a function of age, having a younger population may provide some level of inherent immunity to the coronavirus. Older adults (aged 70+ years) are at increased risk of severe disease and death if they develop COVID-19 whereas pediatric coronavirus disease-19 (COVID-19) infection is relatively mild when compared to adults, and children are reported to have a better prognosis. Mortality in children appears rare [52]. The regressed relationship between age and protection against COVID-19 is depicted in (Figure 4).
Figure 4: Relationship of COVID-19 susceptibility with polyparasitemia. As polyparasitemia increases, susceptibility to SARS-CoV-2 decreases. Intestinal helminths are responsible for the noted polyparasitemia.
Africa’s infectious disease burden
As reported over a decade ago, infectious diseases continue to be the major causes of mortality in Africa [1]. HIV/AIDS and malaria are among the leading causes of morbidity and mortality on the continent [33]. Parasitic infections are widespread throughout the African tropics and sub-tropics, and infection with multiple parasite species is the norm rather than the exception [54]. Well known existing, emerging, and re-emerging diseases like malaria, tuberculosis, HIV/AIDS, cholera, meningitis, hepatitis, schistosomiasis, lymphatic filariasis, trypanosomiasis, Ebola, and other complex diseases that are causing morbidity and mortality to a wide swath of local populations in developing countries in general, and in Africa in particular [55]. The selective burden of these infectious diseases has undoubtedly remodeled the genetic architecture of both the human and parasite populations [56] since the impact of infectious diseases permeates all aspects of African societies, inspiring traditional medicines, producing disabilities, deformities, loss of productivity, increased need for care and treatment, reduced life expectancies, and compromised fertility and fecundity. East and South Africa are perhaps the best studied regions for patterns of parasite loads yet the patterns in these areas may not reflect the worm burdens in other areas of the continent.
Helminthiasis is a major cause of morbidity, especially in resource-limited settings. The incidence of soil-transmitted helminths (STHs) is approximately 50% in developed countries and reaches up to 95% in developing countries, with Sub-Saharan Africa (SSA) having the highest burden of these infections. It is estimated that children or preschool age account for 10–20% of those infected with STHs. The most common of the STH infections are Ascaris lumbricoides infections, Trichuris trichiura infections, and the hookworm infections caused by Necator americanus and Ancylostoma duodenale, In the less-developed countries of Africa, it is common that children are parasitized with more than one species at the same time over the course of their lifetimes. This protracted hyperparasitemia in young children results in impairments in physical, intellectual, and cognitive development [57]. Significantly, these heavy parasite loads tend to affect the immune response of infected children, which may be directly related to their subsequent responses to SARS-CoV-2 exposures.
Regarding death by infectious diseases, Africa is unique in the world; deaths caused by HIV/AIDS, malaria, tuberculosis, and other communicable diseases are predominant. Many Africans sustain multiple infections simultaneously [53] and this polyparasitism may be dramatically changing the environmental context (and specifically the immunological setting) within which SARS-CoV-2 must adapt. Polyparasitism is widespread in many communities in Sub-Saharan Africa [57] with coinfection of malaria and intestinal helminths is among the most common polyparasitic infections. In a recent survey of Nigerian, West African children, a total of 405 children were infected with polyparasitism, 66.17%, 30.12%, and 3.70% for double, triple, and quadruple parasite infections, respectively. Plasmodium falciparum and A. lumbricoides (30.12%); P. falciparum, A. lumbricoides, and T. trichiura (14.81%); and P. falciparum, A. lumbricoides, T. trichiura, and E. histolytica are the most prevalent double, triple, and quadruple parasitic infections recorded [57]. In this West African study, the risk of polyparasitism increased with a child’s age. Figure 5 reflects the regressed relationship between simultaneous infection with multiple intestinal parasites and protection from COVID-19.
Figure 5: Relationship of COVID-19 susceptibility to Age and polyparasitemia. These interactions produce two possible blunted parabolic curves. The optimal response to protection against COVID-19 mortality is with Low chronological age and high polyparasitemia with intestinal helminths. Our model suggests that in the interactions of age + polyparasitemia with helminthiasis, older and low polyparasitemic populations are the most vulnerable to COVID-19 mortality.
Polyparasitism’s Effects on Covid-19
Polyparasitemia refers to the quantitative content of parasites within a host organism and is used as a measure of parasite load. Polyparasitemia is also indicative of the degree of active infection. Multiple parasites concomitantly affect a single host and interact with the host and with each other. The evolutionary question in polyparasitism is how does this protracted history of chronic exposures to a panorama of highly infectious pathogens influence African responses to SARS-CoV-2? Co-infection with intestinal parasites is associated with reduced development of severe COVID-19 in Africa [58]. Could the low incidence of COVID-19 in Africa reflect the confounding effects of endemic malarial parasitemia [59]? The condition of co-infection with multiple parasites may mute hyper-inflammation associated with severe COVID-19, affecting the phenotypes of infected individuals such that, hypothetically, the selective impact of the coronavirus is somewhat thwarted. Specifically, intestinal parasites may stimulate the immunosuppressive and regulatory T-helper 2 (TH2) branch of the immune system, which decreases ACE2-receptor expression. These are the receptors that SARS-CoV-2 preferentially uses to infect host cells. Could polyparasitism balance the inflammatory TH1/TH17 branches of the immune system triggered by SARS-CoV-2 infection? There are shared, common immunodominant epitopes and cross-immunity between COVID-19 and malaria and that give strong evidence as to how malaria and malaria-co-infected individuals might be protected against COVID-19 [59]. Ultimately, Africans exposed to COVID-19 may experience reduced inflammation through the release of anti-inflammatory/regulatory cytokines [60]. Because intestinal helminthic infections are common throughout Africa and affect the most vulnerable and marginalized members of society, it is especially important to consider how these parasites may impact COVID-19 outcomes. Polyparasitism in Africans may thus impact their immunogenetics, their behaviors, and other factors influencing the viability of the coronavirus.
Relationships Between COVID-19 Susceptibility, Age, and Polyparasitism
The reasons for the relative resistance of children to some infectious diseases remains obscure, but it has been suggested [61] that children have a more active innate immune response, healthier respiratory tracts because they have not been exposed to as much cigarette smoke and air pollution as adults, and fewer underlying metabolic disorders. The innate immune system is the first to respond to pathogens and does not retain memory of previous responses and the innate immune responses are evolutionarily older than adaptive immune responses. It may be that among young Africans, youthful age presents a more adverse environment for SARS-CoV-2 proliferation and growth.
Both humoral and cellular immune responses are involved in the pathophysiology of COVID-19 disease, where the principal and effective immune response towards viral infection is the cell-mediated immunity. Humoral immunity and cell-mediated immunity are two types of an adaptive immune response. The immaturity of the infant immune system alters the outcome of viral infections, but at the same time, viral infection shapes the development of the infant immune system and its future responses [63]. Lower levels of cells known as monocytes, including inflammatory monocytes, act as a bridge between the innate and adaptive immune systems. Children appear to mount a less robust adaptive immune response because their innate response was more efficient at eliminating the SARS-CoV-2 threat. Therefore, we hypothesize that it may be this age-associated immunological pliability that is the basis for the positive correlation between age and COVID-19.
Helminth parasites can be tissue dwelling or intestinal, but all induce a dramatic activation and expansion of the Th2 lymphocyte subset. These Th2-derived responses, including IgE, eosinophilia and mastocytosis, are important in the protective immune response to the parasite [60]. Helminth exposure tends to inhibit IFN-γ and IL-17 production, promote IL-4, IL-10, and TGF-β release, induce CD4+ T cell Foxp3 expression, and generate regulatory macrophages, dendritic cells, and B cells [61]. Helminths modulate inflammatory and metabolic pathways in infected humans and polyparasitism may only amplify this response. Therefore, we propose a negative correlation exists between polyparasitism and COVID-19 (as depicted).
When age and parasitemia are combined and contrasted with COVID-19 susceptibilities, two coupled, muted parabolic curves are produced (Figure 5). Mediation of this curve is primarily immunological: The interaction between the SARS-CoV-2 virus and the individual's immune system determines the onset and development of the disease significantly. Both age and parasitemia interact as depicted in Figure 5.
Current Regional Geospatial Distributions of Covid-19 Hot-Spots
As of June 2022, Africa had 518,356 active cases of coronavirus (COVID-19). The top five African countries with high COVID morbidity and mortality span the diverse geospatial regions of the continent. Libya was the leading country, followed by Algeria. The countries reported 75,859 and 58,238 people still infected with the disease, respectively. By the same date, the cumulative number of SARS-CoV-2 in Africa reached more than 8.39 million, while there were 211,853 deaths due to the disease, and 7,661,242 recoveries. The overall patterns of COVID-19 morbidity and mortality different regionally as the disease transitions through diverse geospatial regions of the continent.
SARS-CoV-2 infections and deaths are not uniformly distributed throughout Africa. Within broad geospatial regions of Africa, there are notable country (and presumably ecologically-specific) hot spots right next to adjacent regions with significantly lower frequencies. We can only surmise that the virus is greatly affected by local dynamics and that these regional geospatial differences affect viral transmission dynamics and virulence. The highest number of cases (over 100,000) are currently in South Africa (2.9 million), Morocco (933 thousand), Tunisia (707 thousand), Ethiopia (346 thousand), Libya (340 thousand), Egypt (305 thousand), Kenya (249 thousand), Zambia (209 thousand), Nigeria (206 thousand), Algeria (203 thousand), Botswana (179 thousand), Mozambique (151 thousand), Zimbabwe (131 thousand), Namibia (128 thousand), Ghana (127 thousand), and Uganda (124 thousand). However, these numbers do not consider variations in the total populations of these countries, the regional distributions of their populations, the age structure and presence of comorbidities, and whether each country has reached their predicted country-wide peak in COVID-19 morbidity and mortality.
Regional Anomalies in Uniform Covid-19 Distributions
CDC Africa regularly reports regional variation in COVID-19 morbidity and mortality. From a regional perspective, there appear to be several seeming anomalies where countries with similar ecologies and overlapping ethnicities have vastly different reported levels of SARS-CoV-2 infection and death. On closer inspection, there are some regions of Africa within which there appear to be anomalies (i.e., unusual disparities) in the distribution of the coronavirus, despite often contiguous ecological and ethnographic continuity between regions. These include --
Covid-19 frequency anomalies within the east african cluster
In this part of continental Africa Tanzania has only reached <1% of its peak, yet Kenya is already at 3%, Uganda at 1%, Rwanda at 1%, Burundi at 3%, South Sudan at 5%, while Somali at 10% of its peak, Ethiopia at 7%of its peak, and Eritrea at 24% of its peak. In the United Nations Statistics Division scheme of geographic regions, approximately 16 territories make up Eastern Africa.
COVID-19 frequency anomalies within the central african cluster
In this part of continental Africa, the Democratic Republic of the Congo is already at 9% of its peak, the Republic of the Congo is at 16% of its peak, yet the adjacent country of Zambia is at <1% of its peak, nearby Malawi is at <1%, Zambia is at <1%, yet to the west, Gabon is at 18% of its peak.
COVID-19 frequency anomalies within the north african cluster
Morocco and Western Sahara only show 1% and <1% of their peaks, respectively, yet adjacent Algeria is 11% of peak, Libya is 19% of peak, Egypt is 51% of peak, and Sudan is 14% of its peak. What makes Morocco and Western Sahara so much more behind Egypt, for example, in peaked COVID-19?
COVID-19 frequency anomalies within the south african cluster
In this part of continental Africa, South Africa is already at 23% of its peak, yet the countries that are adjacent to South Africa are much lower: Namibia is at 1%, Botswana is at 2%, Lesotho is at <1%, Esivatini at 1%, Mozambique at <1% and Zimbabwe is at 2% of its peak.
COVID-19 frequency anomalies within the west african cluster
In this part of continental Africa, Mali (24% of its peak), Niger (36% of its peak), Mauritania (23% of its peak), and Burkina Faso are much more similar in COVID-19 infections than to the adjacent West African countries of Nigeria (4% of its peak), Ghana at 4% of its peak, Togo at 2%, Benin at <1%, Ivory Coast at 4%, and Cameroon at 6%.
We are intrigued by the frequent lack of regional continuity in the intensity COVID-19 infections and deaths despite the recency of SARS-CoV-2 in Africa. Are these discrepancies simply a difference in documentation, inherent local biocultural practices, or are other variables at play? In regions with currently low intensities of COVID-19 morbidity and mortality, is this the “calm before the storm”? Can we expect a major intensification of SARS-CoV-2 infections and deaths in the future? Or are we simply seeing some level of inherent protection against severe coronavirus in African populations? The jury is still out on this. It is worth noting, however, that of the African countries nearest their peak of infection and death, the following have reported an increase in the average number of infections over the last 2 weeks: Eritrea, South Africa, Algeria, Democratic Republic of the Congo, and Tanzania.
Future Predictions for Covid-19 in Africa
It has been suggested that West and Central Africa could see a rise in HIV infections and AIDS-related deaths in a few years due to disruptions in health services because of the COVID-19 pandemic. So, although frequencies of COVID-19 morbidity and mortality may not swell, their ecological presence may set the stage for an exacerbation of the lethal consequences of existing infectious and communicable diseases. The recent emergence of the omicron variant (B.1.1.529) of SARS-CoV-2 in southern African nations is potentially ominous. As of this writing, a new variant of coronavirus with multiple unique mutations and apparently high transmissibility has now supplanted the delta variant of SARS-CoV-2 on much of the African continent. This new variant is considered a variant of concern by the World Health Organization, particularly in southern Africa. Here, it appears to be driving new surges of infections and the variants appear to have evolved independently from the delta variant.
Compounding the problems associated with the emergence of new variants of the virus is the continuing issue of underreporting of COVID-19 infections due to under detection in Africa. The World Health Organization notes that only 14.2% of COVID-19 infections are being detected in continental Africa. The major problem may be that very few tests have been administered and the opportunities to become vaccinated with the existing medications are low for the average African. Most Africans do not traditionally go to a health facility when COVID-19-like symptoms appear and these are the locations where testing would occur if it is available. More testing means better detection, faster isolation, less transmission, and more lives preserved. The potential for gaining an accurate account of the magnitude of COVID-19 infection is compromised under these conditions.
SARS-CoV-2 represents a pathogen to which humans have limited to no evolved immune response [60]. If not balanced and controlled, the most severe symptoms of this coronavirus infection are associated with overactive inflammatory immune responses, leading to a cytokine storm, tissue damage, and death. Anti-COVID-19 vaccines could play a huge role in reducing human susceptibilities to the virus. According to WHO, Africa will need more than 900 million vaccine doses to fully vaccinate 40% of its population, yet as of January 10th, 2022 it had procured only 492 million [64]. In December 2021, less than 9% of the African continent was fully vaccinated [64]. The rate limiting factor seems to be the slow pace of providing vaccinations more than vaccine reluctance among the indigenous populations. The inequity in COVID-19 vaccine distribution is prolonging the COVID-19 pandemic, facilitating the emergence of new molecular variants of the virus with the potential for immune evasion, increased disease severity, and further global spread.
We suggest that today, a combination of exculpatory factors, youth, generally low population densities in rural Africa, endemic exposure to helminthiasis, all support an evolutionary medicine hypothesis for COVID-19 in Africa. Chronic intestinal parasites may play an important role in dampening SARS-CoV-2 symptoms and mitigating the worst COVID-19 outcomes, as initially suggested [60]. We have taken this hypothesis a step further and characterized the likely immunological underpinnings of the interrelationships of age and polyparasitism on COVID-19 in Africa. Despite the vaccine nationalism that continues to preclude African access to the vaccines [64], Africa’s underlying human genetic diversity, longstanding exposures to immunologically stimulatory infectious diseases, young age structure, and current willingness to apply available public health measures (e.g., masking, hand washing, social distancing), appear to blunt the catastrophic aspects of COVID-19 endemicity.
Data Availability Statement
All data used in this paper are publicly available through the World Health Organization and CDC-Africa. Data in tables were calculated by the authors based upon publicly available data sites using the most recent data available at the time of this writing.
Conflict of Interest
The authors declare no conflict of interest.
Author Contributions
The paper was conceived and predominantly written by the lead author (FJ) with bibliographic supplementation and editorial input by RF and NG. Illustrations by NG, and HJ. General editorial and technical assistance by Quad Grid Human Analytics Team members KK, JT, and OA."This work is supported by the Division of Engagement and Outreach, All of Us Research Program, National Institutes of Health under award number 1OT20D028395-01."
References
- Boutayeb A. (2010) The impact of infectious diseases on the development of Africa. Handbook of disease burdens and quality of life measures. 2010:1171-88.
- Fenollar F, Mediannikov O. (2018) Emerging infectious diseases in Africa in the 21st century. New Microbes and New Infect. 26(1):S10-S18.
- Ranawaka UK. (2022) Emerging tropical neurological infections. Clin Med (Lond).22(1):18-20.
- EvansMK. (2020) Covid’s Color Line — Infectious Disease, Inequity, and Racial Justice. N Engl J Med. 383(5):408–10.
- Uren C, Möller M, van-Helden PD, Henn BM, Hoal EG, et al. (2017) Population structure and infectious disease risk in southern Africa. Mol Genet Genomics. 292(3):499–509.
- Petersen DC, Steyl C, Scholtz D, Baker B, Abdullah I, et al. (2022) African Genetic Representation in the Context ofSARS-CoV-2 Infection and COVID-19 Severity.FrontGenet.13:909-117
- Choudhary S, Sreenivasulu K, Mitra P, Misra S, Sharma P, et al. (2021) Role of Genetic Variants and GeneExpression in the Susceptibility and Severity of COVID-19. Ann Lab Med. 1(2):129-38
- Khalid Z, Naveed H. (2022) Identification of destabilizing SNPs in SARS-CoV2-ACE2 protein and spike glycoprotein: implications for virus entry mechanisms. J Biomol Struct Dyn. 40(3):1205-15.
- AlKuraishy MH,Hussain NR, AlGareeb IA, Harchan NA, AlKurashi HA, et al. (2020) The Potential Role of Renin Angiotensin System(RAS) and Dipeptidyl Peptidase-4 (DPP4) in COVID-19: Navigating the Uncharted. IN SelectedChapters from the Renin-Angiotensin System. 10:57-72
- Hoffmann M, Kleine-Weber H, Schroeder S,KrügerN ,Herller T, et al. (2020) SARS-CoV-2 cell entry depends on ACE2and TMPRSS2 and is blocked by a clinically proven protease inhibitor. Cell. 181(2):271-80
- Bunyavanich S, Grant C, Vicencio A. (2020) Racial/Ethnic Variation in Nasal Gene Expression of Transmembrane Serine Protease 2 (TMPRSS2). JAMA.324(15):1567–68.
- Drosten C, Schorder S, kruger N, Herller G, Nitsche A, et al. (2013) Dipeptidyl peptidase 4 is a functional receptor for the emerginghuman coronavirus-EMC. Nature. 495(7440):251–4
- Williams SM, Sirugo G, Tishkoff SA. (2021). Embracing African Genetic Diversity Med. 2(1):19–20
- Graham RL, BaricRS. (2010) Recombination, reservoirs, and the modular spike: mechanisms of coronavirus cross-species transmission. J Virol. 84(7):3134–46.
- Daneshpajouhnejad P, Kopp JB, Winkler CA, Rosenberg AZ. (2022) The evolving story of apolipoprotein L1 nephropathy: the end of the beginning. Nat Rev Nephrol. 18(5):307-20.
- Popovici J, Roesch C, RougeronV. (2020) The enigmatic mechanisms by which Plasmodium vivax infects Duffy-negative individuals. PLOS Pathogens. 16(2):e1008258
- Ahmed JS, GuyahB, SangD, WebaleMK, Mufyongo NS, Munde E, Ouma C.(2020)Influence of blood groupGlucose-6-phosphate dehydrogenase and Haemoglobin genotype on Falciparum malaria in children in Vihiga highland of Western Kenya. BMC infectious diseases. 20(1): 487.
- BoubaYT, sinda EK, Fonkou MDM, Mmbando GS, BragazziNL, Kong JD. (2021) The Determinants of the Low COVID-19 Transmission and Mortality Rates in Africa: A Cross-Country Analysis. Frontiers in Public Health. 9:751197.
- Ibn-Mohammed T, Mustapha KB, Godsell J, Adamu Z, Babatunde KA, Akintade DD, et al. (2021)A criticalanalysis of the impacts of COVID-19 on the global economy and ecosystems and opportunitiesfor circular economy strategies. Resour Conserv Recycl. 164:105169.
- Wale-Oshinowo BA, Omobowale AO, Adeyeye MM, Lebura S. (2020) Least Developed Countries in Africa. The Palgrave Encyclopedia of Global Security Studies. 1–16.
- Lone SA, Ahmad A. (2020) COVID-19 pandemic - an African perspective. Emerg Microbes Infect. 9(1):1300-1308.
- Bassetti M, Vena A, Giacobbe DR. (2020) The novel Chinese coronavirus (2019-nCoV) infections: challenges for fighting the storm. Eur J Clin Invest.50(3) :13209.
- Ji W, Wang W, Zhao X, Zai J, Li X. (2020) Cross-species transmission of the newly identified coronavirus 2019-nCoV. J Med Virol. 92(4):433–440.
- Lu H, Stratton CW, Tang Y. (2020) Outbreak of pneumonia of unknown etiology in Wuhan, China: themystery and the miracle. J Med Virol.92(4):401–402.
- Mallapaty S. (2022) Kids and covid: Why young immune systems are still on top. Nature News 597(7875):166-68.
- Ren LL, Wang YM, Wu ZQ, Xiang ZC, Guo L, Xu T,et al. (2020) Identification of a novel coronavirus causing severe pneumonia in human. Chinese Med J. 133(9):1015–24.
- Rothan HA, Byrareddy SN. (2020) The epidemiology and pathogenesis of coronavirus disease (COVID-19) outbreak. J Autoimmun.109:102433.
- Elias C, Sekri A, Leblanc P, Cucherat M, Vanhems P. (2021) The incubation period of COVID-19: Ameta-analysis, International Journal of Infectious Diseases.Int J Infect Dis. 104:708-10 .
- Homma Y, Katsuta T, Oka H, Iwaki H, Yamashita Y, Shinomiya H, et al. (2021) he incubation period of theSARS-CoV-2 B1.1.7 variant is shorter than that of other strains. Journal of Infection. 83(2):15-17.
- Cao Yu, Chen M, Dong D, Xie S, Liu M. (2020) Environmental pollutants damage airway epithelial cell cilia: implications for the prevention of obstructive lung diseases. Thoracic Cancer. 11(3):505–10.
- Kucharski AJ, Russell TW, Diamond C, Liu Y, Edmunds J, et al. (2020) Early dynamics of transmission and control of COVID-19: a mathematical modeling study. Lancet Infect. 20(5):553–58.
- Wang D, Hu B, Hu C, Zhu F, Liu X, et al. (2019) Clinical characteristics of 138 hospitalized patients with 2019novel coronavirus–infected pneumonia in Wuhan, China. J Am Med Assoc.323(11):1061-69.
- Wang L, Wang Y, Ye D, Liu Q. (2020) Review of the 2019 novel coronavirus (SARS-CoV-2) based on current evidence. Int J Antimicrob Agents.55(6):105948.
- Wang W, Tang J, Wei F. (2020) Updated understanding of the outbreak of 2019 novel coronavirus(2019-nCoV) in Wuhan, China. J Med Virol.92(4):441–47.
- Assiri A, Al-Tawfiq JA, Al-Rabeeah AA, Al-Rabiah FA, Al-Hajjar S, et alEpidemiological, demographic, and clinical characteristics of 47 cases of Middle East respiratory syndrome coronavirus disease from Saudi Arabia: a descriptive study. Lancet Infect. Dis. 13(9):752–61.
- Carlos WG, Dela Cruz CS, Cao B, Pasnick S, Jamil S, et al. (2020) Novel wuhan (2019-nCoV) coronavirus. Am. J. Respir. Crit Care Med. 201(4):7-8.
- Chan JFW, Yuan S, Kok K-H, Chu H, Yang J, et al. (2020) A familial cluster of pneumonia associated with the 2019 novel coronavirus indicating person-to-person transmission: a study of a family cluster. Lancet. 395(10223):514–23.
- Li Q, Guan X, Wu P, Wang X, Zhou L, et al. (2020) Early transmission dynamics in Wuhan, China, of novel coronavirus–infected pneumonia. N Engl J Med. 382:1199–1207.
- Li R, Pei S, Chen B, Song Y, Zhang T, et al. (2020) Substantial undocumented infection facilitates the rapid dissemination of novel coronavirus (SARS-CoV-2) Science. 368(6490):489–93.
- Li X, Xu S, Yu M, Wang K, Tao Y, et al. (2020) Risk factors for severity and mortality in adult COVID-19 inpatients in Wuhan. J Allergy Clin Immunol.146(1):110–118.
- Ghinai I, McPherson TD, Hunter JC, Wang C, lindstorm s, et al. (2020) First known person-to-person transmission of severe acute respiratory syndrome coronavirus 2 (SARS-CoV-2) in the USA. Lancet. 395(10230):1137–44.
- Huang C, Wang Y, Li X, Ren L, Zhao J, et al. (2020) Clinical features of patients infected with 2019 novelcoronavirus in Wuhan, China. Lancet. 395(10223):497–506.
- Huang R, Zhu L, Xue L, Liu L,Wang J, et al. (2020) Clinical findings ofpatients with coronavirus disease 2019 in Jiangsu province, China: a retrospective, multi-centerstudy.PLoSNegl Trop Dis. 14(5):e0008280.
- Yu YX, Sun L, Yao K, Lou XT, Liang X, et al. (2020) [Consideration and prevention for the aerosoltransmission of 2019 novel coronavirus][Zhonghuayanke za zhi] Chinese journal of ophthalmology.Zhonghua Yan Ke Za Zhi. 56(9):653-56.
- Fang L, Karakiulakis G, Roth M. (2020) Are patients with hypertension and diabetes mellitus at increased risk for COVID-19 infection? The Lancet Respiratory Medicine.Lancet Respir Med. 8(4):e21.
- Giannis D, Ziogas IA, Gianni P, et al. (2020) Coagulation disorders in coronavirus infected patients: COVID-19, SARS-CoV-1, MERS-CoV and lessons from the past. J Clin Virol. 127:104362.
- Wu JT, Leung K, Leung GM, et al. (2020) Nowcasting and forecasting the potential domestic and international spread of the 2019-nCoV outbreak originating in Wuhan, China: a modeling study. Lancet. 395(10225):689–97.
- Xiao F, Tang M, Zheng X, Liu Y, Li X, et al. (2020) Evidence for gastrointestinal infection ofSARS-CoV-2. Gastroenterology.158(6):1831–33.
- Zhou F, Yu T, Du R, Fan G, Liu Y, et al. (2020) Clinical course and risk factors for mortality of adult inpatients with COVID-19 in Wuhan, China: a retrospective cohort study. Lancet. 395(10229):1054–62.
- www.statista.com/statistics/265759/world-population-by-age-and-region/
- Khayat AS, de Assumpcao P, Khayat BCM, Araujo TMT, Batista-Gomes A, et al. (2020) ACE2 polymorphisms as potential players in COVID-19 outcome. PLoS One. 15(12):e0243887.
- Balasubramanian S, Rao NM, Goenka A, Roderick M, Ramanan AV. (2020) Coronavirus Disease 2019 (COVID-19) in Children - What We Know So Far and What We Do Not. Indian Pediatr. 15;57(5):435-42.
- Pullan R, Brooker S. (2008) The health impact of polyparasitism in humans: are we under-estimating the burden of parasitic diseases? Parasitology. 135(7):783-94.
- Allen JE, Maizels RM. (1996) Immunology of Human Helminth Infection. Int Arch Allergy Immunol.109(1):3-10.
- https://www.scirp.org/%28S%28vtj3fa45qm1ean45vvffcz55%29%29/reference/referencespapers.aspx?referenceid=3039373
- Chapman SJ, Hill AVS. (2012) Human genetic susceptibility to infectious disease. Nat Rev Genet 13(3):175-88.
- Odoemene SN, Oluwole AS, Mogaji HO, Adegbola MV, Omitola OO, et al. (2020) Polyparasitism with Malaria and Intestinal Parasite Infections among Infants and Preschool-Aged Children in Egbedore, Osun State, Nigeria. J Parasitol Res.
- Wolday D, Gebrecherkos T, Arefaine ZG, Kiros YK, Gebreegzabher A, et al. (2021) Effect of co-infection with intestinal parasites on COVID-19 severity: A prospective observational cohort study. E Clinical Med. 39:101054.
- Osei SA, Biney RP, Anning AS, Nortey LN, Ghartey-Kwansah G. (2022) Low incidence of COVID-19 case severity and mortality in Africa; Could malaria co-infection provide the missing link?. BMC Infect Dis. 22(1):78.
- Cepon-Robins TJ, Gildner TE. (2020) Old friends meet a new foe: A potential role for immune-priming parasites in mitigating COVID-19 morbidity and mortality. Evol Med Public Health. 22(1):78.
- Lee PI, Hu YL, Chen PY, Huang YC, Hsueh PR. (2020) Are children less susceptible to COVID-19? J Microbiol Immunol Infect. 53(3):371-72.
- Elliott DE, Weinstock JV. (2021) Helminth–host immunological interactions: prevention and control of immune-mediated diseases. Ann N Y Acad Sci.1247:83-96.
- Tregoning JS, Schwarze J. (2010) Respiratory viral infections in infants: causes, clinical symptoms, virology, and immunology. Clin Microbiol Rev.23(1):74-98.
- The Lancet Infectious Diseases. (2022) Time for Africa to future-proof, starting with COVID-19. Lancet Infect Dis. 22(2):151.