Potential of Stem Cell Based Therapy to Treat Celiac Disease and its Complications
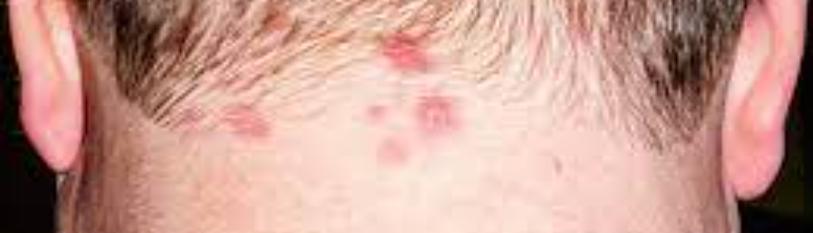
Hetvi Solanki, Vincent S. Gallicchio*
Department of Biological Sciences, College of Science Clemson University Clemson, SC, USA 29636
*Corresponding author: Vincent S. Gallicchio. Department of Biological Sciences, 122 Long Hall College of Science, Clemson University, Clemson, South Carolina, USA, 2963
Citation: Solanki H, Gallicchio VS. (2022) Potential of Stem Cell Based Therapy to Treat Celiac Disease and its Complications. J Stem Cell Res. 4(1):1-7.
Received: December 7, 2022 | Published: January 26, 2023
Copyright© 2023 genesis pub by Solanki H, et al. CC BY NC-ND 4.0 DEED. This is an open-access article distributed under the terms of the Creative Commons Attribution-NonCommercial-No Derivatives 4.0 International License., This allows others distribute, remix, tweak, and build upon the work, even commercially, as long as they credit the authors for the original creation.
DOI: https://doi.org/10.52793/JSCR.2023.4(1)-43
Abstract
Celiac Disease affects 1% of the population in the Western world, however, the number of affected people is rising due to high gluten diets. Celiac disease is caused by a number of things involving interactions between genetic, environmental, and immunological factors. Lack of a comprehensive model to conduct trials on makes treating celiac disease a difficult feat. In terms of clinical trials, low incidence and variability in patients are significant obstacles in the establishment of treatments. Stem cells play a pivotal role in chronic inflammatory pathologies due to their ability to regenerate and differentiate into numerous cell types as well as their essential role in homeostasis. Mesenchymal stem cells are highly proliferating, multipotent stromal cells that can cross the HLAQ barrier and lack immunogenicity. Based on these properties, mesenchymal stem cells are able to maintain the epithelial barrier, prevent villous atrophy, and subdue inflammation in CD patients and have proven to be successful in multiple clinical trials. For this reason, stem cells, primarily mesenchymal, possess the potential to be an effective therapy and should be further researched.
Keywords
Celiac Disease; Stem cells; Mesenchymal stem cells; Hematopoietic stem cells
Introduction
Celiac disease is caused by several things involving interactions between genetic, environmental, and immunological factors [1]. Genetic predispositions for this disease are caused by heterozygous expression of HLA-DQ2 and HLA-DQ8 genes. The disease in its inactive form is mainly triggered by gluten peptides [2]. A delay in the diagnosis of celiac disease may lead to an increased risk of malignant complications. Celiac disease can progress into malignant complications such as refractoriness and enteropathy-associated T-cell lymphomas (EATL). Traditional treatment methods for CD include a gluten-free diet (GFD), cyclophosphamide-, adriamycin-, vincristine-, and prednisone (CHOP) based chemotherapy with or without ASCT, and surgical removal of EATLs, however, these treatments do not display promising outcomes [2]. CHOP-based chemotherapy with or without autologous stem cell transplant (ASCT) has a significant level of morbidity associated with it and has not been overall successful enough to be considered as a standard of treatment. Surgical removal of EATLs is invasive and often seen as a last resort [3]. Gluten-free diets have been designated as the first approach for patients with Celiac Disease. However, GFDs have a history of low compliance due to cost and social pressures [4]. GFDs can also significantly reduce the quality of life due to the limited number of options available and the possibility of cross-contamination [5].
Stem cells play a pivotal role in chronic inflammatory pathologies due to their ability to regenerate and differentiate into numerous cell types as well as their essential role in homeostasis. Hematopoietic stem cells and mesenchymal stem cells are the two types of stem cells with the greatest potential of differentiating into cells of diverse tissue types [1]. Mesenchymal stem cells are highly proliferating, multipotent stromal cells that can cross the HLAQ barrier, and their lack of immunogenicity make MSCs more promising than HSCs as a therapy for celiac disease. For this reason, stem cells, primarily mesenchymal, possess the potential to be an effective therapy and should be further researched [2].Hematopoietic stem cell transplant has been successful in allowing CD patients to develop gluten tolerance[6]. Mesenchymal stem cells can maintain the epithelial barrier, prevent villous atrophy, and subdue inflammation in CD patients and have proven to be successful in multiple clinical trials [2]. This literary review summarizes current research on the application of stem cell-based therapy as a method of treatment for celiac disease including the pathophysiology and causes of celiac disease, the current standard of care, why treatments have failed, the role of mesenchymal stem cells within the digestive system as a treatment for CD, and promising results from clinical trials.
Epidemiology
Celiac Disease affects 1% of the population in the Western world, however, the number of affected people is rising due to high gluten diets [4]. Complicated cases such as refractory celiac disease occur in 2-5% of patients [2]. EATLs, which are aggressive T-cell lymphomas arising in the GI tract due to refractory celiac disease, predominantly affect males in their 60s and 70s [3].
Pathophysiology
Celiac disease involves interactions between genetic, immunological, and environmental factors [1]. Genetic susceptibility for CD is due to variants of HLA class II genes (HLA-DQ2.5, HLADQ2.2, HLADQ8) and carriership. Environmental factors that contribute to CD include ATIs (amylase trypsin inhibitors) found in gluten that can trigger Toll-like receptor 4-dependent innate immune response in the small intestine, viral infections, and an altered gut microbiome [5]. CD-associated bacteria produce shorter gluten peptides that can easily cross the intestinal epithelial barrier and activate gluten-specific T-cells [5]. Observations suggest primary barrier defect is the reason CD occurs [5]. In normal patients, the GI tract is regularly exposed to harsh chemical and mechanical conditions, due to this the tract has a self-renewal process. In patients with celiac disease, this self-renewal process does not function correctly [1].
CD is diagnosed based on a serological test for atTG antibodies, EMA antibodies, a biopsy, and an endoscopy of the small intestine [7]. There are three types of CD: subclinical which is asymptomatic, non-clinical in which unrelated symptoms are presented, and classical in which the patient exhibits severe symptoms [7]. In patients with active CD, phosphorylation, mediated by pro-inflammatory cytokines, is altered at tight and adherens junctions [8]. In the early diagnosis of CD, the permeability of the epithelial barrier is increased due to gliadin-dependent activation of the zonulin pathway in enterocytes which leads to cytoskeleton reorganization and the tight junction opening [8]. Gluten proteins contain large amounts of glutamine and proline residues which are difficult to digest [5]. Undigested proteins pass through the epithelial barrier and enter the lamina propria where they are deamidated by TG2. This process eventually leads to villous atrophy [5]. Both innate and adaptive immune responses cause intestinal lesions in patients with CD and lymphomagenesis in refractory CD [8].
Refractory CD occurs in 2-5% of patients [2]. There are two types of RCD. In RCD1, patients develop enteropathy-associated T-cell lymphomas (EATLs) [2]. In RCD2 patients have surfaces of CD8 and CD3 that lack expression leading to a cryptic lymphoma [2]. In patients with refractory CD, IL-15 promotes T-cell cytotoxicity and is overexpressed in celiac mucosa [3]. This overexpression leads to effector T-cells being resistant to CD4+ CD25+ transcription factor (FOXP3+) and inhibits regulatory T-cells from suppressive activity [2]. Unacceptable IEL levels are a result of IL -15 [2].By activation of JAK3 and STAT5, IL15 induces anti-apoptotic BCL to be expressed, leading to IEL being unable to perform apoptosis and progress malignantly [2]. In patients with EATLs: anemia, abnormal WBC count, elevated LDH (lactate dehydrogenase), ESR (erythrocyte sedimentation rate), CRP (C-reactive protein) and decreased albumin are lab abnormalities that are present [3]. Modifications in key epithelial signaling pathways cause many immature epithelial cells that eventually lead to epithelial dysfunction, promote crypt hyperplasia, and increase intestinal permeability [9]. This leads to immune cells having greater access to gluten and causing a proinflammatory immune response and positive feedback loop responsible for epithelial damage [9].
Why treatments have failed
While many treatments for celiac disease have been attempted, not many have succeeded. Larazotide acetate has been proposed as a potential therapy due to its role as a zonulin antagonist thus keeping tight junctions in the epithelium closed. While GI symptoms improved when this treatment was implemented, no change was observed in intestinal permeability despite two double-blind, randomized, placebo-controlled, clinical trials being conducted [8]. Hemopoietic stem cell transplantation possesses the ability to regenerate gut mucosa and reset the immune system. However, when implemented in a clinical trial many relapses occurred and the risk for mortality was too high to consider it as a treatment for non-life-threatening celiac disease [2]. In addition, no model can fully capture the complexity of Celiac Disease [5]. Many models have been attempted but each has its own flaws. IECs do not consider intestinal physiology [5]. Biopsies are invasive and have limited ability to proliferate or be studied [5]. In vitro studies regarding the role of gliadin toxicity in the pathogenesis of celiac disease have all been 2D [10]. The lack of a comprehensive model to conduct trials on makes treating celiac disease a difficult feat. In terms of clinical trials, low incidence and variability in patients are significant obstacles in the establishment of treatments [3]. Overall, more information in many areas such as stem cell harvesting protocols and apoptosis regulation is needed to establish an effective therapy for celiac disease [11-12].
Discussion
Stem cells play a pivotal role in chronic inflammatory pathologies due to their ability to regenerate and differentiate into numerous cell types as well as their essential role in homeostasis. Hematopoietic stem cells and mesenchymal stem cells are the two types of stem cells with the greatest potential of differentiating into cells of diverse tissue types [1]. Mesenchymal stem cells are highly proliferating, multipotent stromal cells that lack immunogenicity, which makes them a promising therapy for CD [2]. Numerous human clinical provide evidence that MSCs can target nearly all mechanisms involved in CD pathogenesis [2].
Use of stem cells (especially mesenchymal) to treat celiac disease
Mesenchymal stem cells possess three criteria: they are plastic adherent under standard culture conditions, they differentiate into adipocytes, osteoblasts, and chondroblasts in vitro, and they express CD105, CD73, and CD90 and lack surface expression of CD45, CD34, CD14, CD11b, CD19, and HLA-DR. Mesenchymal stem cells’ lack of immunogenicity makes MSCs more promising than HSCs as a therapy for celiac disease [2]. MSCs possess a strong modulatory effect on all immune cells with a possible regenerative effect and are more suitable for transplant [2]. MSCs possess greater suitability for transplant because they can cross HLQA barriers due to their lack of expression of MHC class 2 and minimal expression of MHC class 1 antigens as well as the absence of CD40, CD80, and CD86[2]. MSCs create a quasi-niche due to their anti-inflammatory and modulatory properties that prime naïve immune cells towards a tolerogenic profile [2]. The expression of HLA-G allows MSCs to inhibit the proliferation of CD8+T cells, suppress NK cells’ lytic activity, and expand regulatory T-cells[2]. In addition, patients with RCD will be protected from developing EATLs by the inhibitory function of MSC on IL-15 secretion and function [2]. MSCs also possess the ability to resemble claudins which allows them to maintain the epithelial barrier [2]. Villous atrophy can be prevented by MSCs as well. MSCs can secrete IL6, HGF, and VEGF to inhibit the interaction of FAS receptors with their ligands. This will then prevent the activation of caspase-3 and caspase-8 which are responsible for the apoptosis of enterocytes that lead to villous atrophy [2]. MSCs are vulnerable to lysis by IL2-activated NKs, however, due to excessive IFN-y found in the celiac mucosa, a favorable microenvironment for MSCs is created [2]. MSCs can block the G0 to G1 phase progression of monocytes thus preventing them from differentiating into dendritic cells [2]. MSCs can also secrete IL6, PGE2, and CSF which can turn mature dendritic cells into less mature phenotypes that express CD40, CD80, CD83, CD86, and HL class 2 molecules, less on their surface. MSCs shifting these dendritic cells towards a more tolerogenic profile can potentially avoid the activation of T-cells and subdue inflammation in CD [2]. TNF-a down regulation by MSCs can prevent CD patients from developing severe complications [2]. Overall, MSCs can target nearly all mechanisms involved in CD pathogenesis. Limitations are that they only act in specific mucosal environments, they must be obtained from sources that have unlimited donors such as umbilical cord blood, amniotic fluid, or the placenta, and they can get trapped in the lungs because of their large size [2] (Figure 1).
Figure 1: MSCs’ interaction with all of the immune cells involved in CD pathogenesis: B-cells, regulatory T-cells, T lymphocytes, and endothelium, is depicted. Cell-to-Cell interaction, different factors and chemokines like FoxP3, HLA G, IFN Y, PGE2, CSF, CCL7, IDO, and IL16 as well as claudin for reassembling tight junctions, are the basis of the inhibitory effects of MSCs [13].
Results of clinical trials and why/why not they were effective
When MSCs were infused into mice with Type 1 diabetes, they prevented the insulin-producing beta cells in the pancreas from being attached as well as suppressed diabetes-specific T-cell proliferation and IFN-y production. Since CD and Type 1 diabetes are similar in pathophysiology, MSCs are expected to work in a similar way on gliadin-specific T cells in patients with CD [8].
In another clinical trial, 26 CD patients underwent chemotherapy consisting of IVE/MTX (ifosfamide, vincristine, etoposide, and methotrexate) followed by ASCT and promising results were produced. A superior ORR compared to an anthracycline-based regiment (69% vs 42%) was observed. Only 19% of patients discontinued chemotherapy. 67% of patients underwent ASCT which resulted in improved 5-year progression-free-survival. Overall survival rates of 52% and 60% were observed along with a decreased death rate. This clinical trial had an acceptable safety profile [3].
In 44 CD patients, ASCT with a 46-month follow-up was conducted. The relapse rate over four years was found to be 39%. The progression-free-survival rate was found to be 54% and the overall survival rate was found to be 59%. The survival curve plateaued around 30 months which suggests that this treatment could be a possible cure. Rates of relapse decreased after 18 months however, a 2-year-non-relapse mortality rate of 7% can be viewed as a concern. Intensive IVE/MTX regimen followed by ASCT led to a superior 5-year OS of 60% in comparison with historical controls of 22%, a superior PFS value of 52% compared to 22% historically, and decreased mortality with only 39% versus 81%. ASCT seems to produce favorable outcomes and should be considered in patients that are newly diagnosed with EATL and eligible for a transplant [3].
In a 61-year-old female with fatal malabsorption from steroid-refractory autoimmune enteropathy, MSC transplant proved to be successful. She underwent two infusions of bone marrow MSCs. Within a month, serologic and histologic landmarks of the disease disappeared. An increase in mucosal FoxP3+ Tregs and secretory immunoglobulins was observed. Considering the similarities between steroid-refractory autoimmune enteropathy and celiac diseases, MSC transplant has the potential to be successful in CD patients as well [8].
A 51-year-old female with type II RCD was successfully treated with MSC. It was confirmed that the patient followed a GFD for 14 years post-CD-diagnosis. The patient reported severe diarrhea, fever, weight loss, dehydration, and electrolyte imbalance. A course of total parenteral nutrition with antibiotic and antifungal drug therapy was conducted following the isolation of staph epidermis in blood culture and E. coli in bronchoalveolar lavage fluid and Candida albicans in the stool. atTG and anti-enterocyte antibodies were negative. The patient was initially treated with budesonide for refractoriness which produced good results after 5 years. 50 mL of bone marrow blood was harvested and 3.9x cells matched all the MSC identification criteria. MSCs were isolated, expanded, and characterized which is a part of standard protocol. The patient’s malabsorption index, mucosal architecture, and % of aberrant intraepithelial lymphocytes were measured at enrollment, infusions, and 6 months. The mucosal expression of IL-15 and its receptor was measured and MSC expansion was deemed as feasible. 4 systemic infusions of 2 x MSCs/kg body weight were administered 4 months apart with no observable adverse effects. Serial infusions were used because of previous evidence of a loss of efficacy in an adult autoimmune enteropathy case which could also be explained by the short life span of MSCs in vitro. Within a month, normalization began occurring; the critical stage was overcome, mucosal architecture recovered, and slower progressive absorptive capacity was found. 6 months after the treatment ended, a full macro and microscopic recovery were observed. The patient seemed to experience improvement based on the observation that stool frequency, BMI, lab tests, and mucosal architecture were all normalized. The expression of IL-15 and its receptor mostly disappeared. After 6 months, matters of concern were scars at the wireless capsule endoscopy site and aberrant IELs> 90% as well as monoclonal rearrangement of beta and gamma chains were still present [14].
2 patients with CD and be tathalassemia major were studied. Both underwent successful myeloablative allogenic HSCT for beta thalassemia major. Following HSCT, gluten was reintroduced into the patient’s diet. Following the reintroduction of gluten, no clinical, serological, or histological markers of CD arose for 5 years. T-cells did not proliferate upon gliadin stimulation and dendritic cells and FoxP3T cells displayed normal values. The acquired data suggests that allogeneic HSCT may allow CD patients to acquire gluten tolerance. No other therapies have done this before; in most cases, the patient experiences a relapse within 2 years of the reintroduction of gluten. However, HSCT is not successful in treating RCD or EATLs [6].
Conclusion
Interactions between genetic, immunological, and environmental factors all contribute to the development of CD [1]. The incidence of CD is increasing in the Western world due to high gluten diets [4]. In patients with CD, the GI tract’s process of self-renewal is flawed [1]. A delay in the diagnosis of celiac disease may lead to an increased risk of malignant complications. Celiac disease can progress into malignant complications such as refractoriness and enteropathy- associated T-cell lymphomas (EATL).Traditional treatment methods for CD include a gluten-free diet (GFD), cyclophosphamide-, adriamycin-, vincristine-, and prednisone (CHOP) based chemotherapy with or without ASCT, and surgical removal of EATLs, however, these treatments do not display promising outcomes [2].Based on the properties of stem cells, especially mesenchymal, stem cell therapy seems to be a promising treatment for chronic inflammatory pathologies like CD [1,2,4]. However, first and foremost, a comprehensive model of CD is needed because without a model that is fully able to capture the complexity of CD, developing therapies is a difficult feat [5]. In addition, while several clinical trials provide evidence that MSCs can target nearly all mechanisms involved in CD pathogenesis, more clinical trials need to be conducted to further support this hypothesis [1].
References
- Piscaglia, A. C. (2014). Intestinal Stem Cells and Celiac Disease. World J Stem Cells, 6(2):213-29.
- Moheb-Alian A, Forouzesh F, Rostami-Nejad M, Rostami K. (2016). Mesenchymal stem cells as potential therapeutic approaches in celiac disease. Gastroenterol hepatol bed bench. 9(Suppl1):S1–S7.
- Ondrejka S, Jagadeesh D. (2016). Enteropathy-associated T-cell lymphoma. Curr Hematol Malig Rep. 11(6):504–13.
- Lerner A. (2012). Future Therapeutics in Celiac Disease. Harefuah. 151(6):358–77.
- Moerkens R, Mooiweer J, Withoff S, Wijmenga C. (2019). Celiac Disease‐on‐Chip: Modeling a Multifactorial Disease in vitro. United European Gastroenterology J. 7(4): 467–76.
- Ciccocioppo R, Bernardo ME, Russo ML, Vanoli A, Franco C, et al. (2013). Allogeneic Hematopoietic Stem Cell Transplantation May Restore Gluten Tolerance in Patients with Celiac Disease. J Pediatr Gastroenterol Nutr. 56(4):422–27.
- Piscaglia AC, Rutella S, Laterza L, Csario V, Campanale M, et al (2015). Circulating Hematopoietic Stem Cells and Putative Intestinal Stem Cells in Coeliac Disease. J Transl Med. 13(1):220.
- Ciccocioppo R, Cangemi GC, Roselli EA, Kruzliak P. (2014). Are Stem Cells a Potential Therapeutic Tool in Coeliac Disease? Cell Mol Life Sci. 72(7):1317–29.
- Escudero-Hernández C. (2021). Epithelial Cell Dysfunction in Coeliac Disease. Int Rev Cell Mol Biol, 133–164.
- Dolfini E, Elli L, Ferrero S, Braidotti P, Roncoroni L, et al. (2003). Bread wheat gliadin cytotoxicity: A new three‐dimensional cell model. Scand J Clin Lab Invest. 63(2):135–42.
- Lacob R, Sîrbu-Boeţi P, Iacob S, Dima S, Gheorghe C, et al (2009). Stem cells therapies for gastrointestinal and liver diseases. Chirurgia (Bucur). 104(2):131–40.
- Ciccocioppo R, Di Sabatino A, Gasbarrini G, Corazza GR. (1999). Apoptosis and gastrointestinal tract. Ital j gastroenterol hepatol. 31(2):162–72.
- Moheb-Alian A, Forouzesh F, Rostami-Nejad M, Rostami K. (2016). Mesenchymal stem cells as potential therapeutic approaches in celiac disease. Gastroenterol hepatol bed Bench. 9(Suppl1):S1–S7.
- Ciccocioppo R, Gallia A, Avanzini MA, Betti E, Picone C,et al (2016). A refractory celiac patient successfully treated with mesenchymal stem cell infusions. Mayo Clinic Proc. 91(6):812–19.