New Admixture Patterns Trigger Gene-Environment Mismatch between APOL1 Risk Alleles and Chronic Kidney Disease Disparities
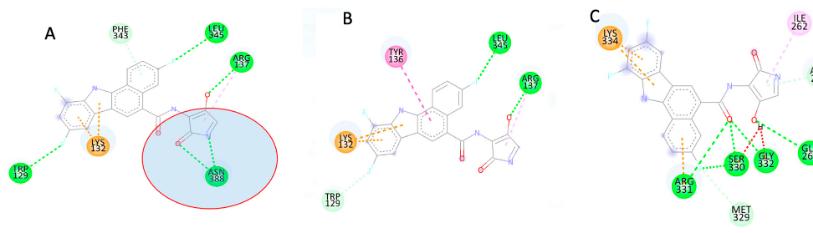
Fatimah LC Jackson1,2*, Nicholas Guthrie1,4, Raven Flowers1,2, Shireen Shah1,3, Vick Mahase1,2, Hasan Jackson1, and Quad Grid Human Analytics Research Team1
1QuadGrid Data Lab, Bethesda, MD
2 Howard University, Washington, DC
3 University of Virginia, Charlotte, VA
4George Washington University, Washington, DC
*Corresponding author: Fatimah LC Jackson, Quad Grid Data Lab, Bethesda, MD
Citation: Jackson FLC, Guthrie N, Flowers R, Shah S, Mahase V, Jackson H. (2023) New Admixture Patterns Trigger Gene-Environment Mismatch between APOL1 Risk Alleles and Chronic Kidney Disease Disparities. Adv Clin Med Res. 4(4):1-23.
Received: August 14, 2023 | Published: September 14, 2023
Copyright© 2023 genesis pub by Jackson FLC, et al. CC BY-NC-ND 4.0 DEED. This is an open-access article distributed under the terms of the Creative Commons Attribution-Non Commercial-No Derivatives 4.0 International License., This allows others distribute, remix, tweak, and build upon the work, even commercially, as long as they credit the authors for the original creation.
DOI: https://doi.org/10.52793/ACMR.2023.4(4)-65
Abstract
The underlying evolutionary genetics of African Americans in North America increases their risk for chronic kidney disease and accounts, in part, for the significant health disparity observed. In this paper we suggest that a gene-environment mismatch is responsible for the high frequency of chronic kidney disease among many African Americans and that this mismatch is augmented by gene-gene interactions that enhance the pathology proliferates in a specific environmental setting of high infectious disease (specifically trypanosomiasis) and then loses its evolutionary advantage when the population is abruptly transferred to a new environment (North America) lacking these specific selective constraints. The inordinately high frequencies of chronic kidney diseases in this evolutionarily new environment are a direct result of a mismatch between the group’s background genetics, adaptations to their environments of origin, and the recent migratory transition to the Americas with new selective constraints coupled with the interactions of the original genetic adaptations with other nearby genetic and non-genetic traits. From historic demographic perspectives, the high level of kidney disease in African Americans is also a consequence of the robust interregional African-African genetic admixtures associated with ancestral relocations to the Americas and the resulting mating patterns that (intentionally) merged geographical groups of Africans who had been previously separated. Consequently, the potential developed for the compound double heterozygotes for the APOL1 genetic variants G1 and G2 with an increased risk for CKD has become elevated among the transatlantic African Diaspora populations.
Keywords
African Americans; G1/G2 compound double heterozygote; Selection dynamics; Microevolutionary shifts.
Lay Summary
Centuries of unique interregional African-African genetic admixtures in the Americas have set the stage for the increased contact between Africans with ancestries from different regions of the continent and the increased incidence of producing compound heterozygote’s for APOL1. This phenotype is associated with damage to the kidney.
Introduction
Chronic kidney disease (CKD) is a global health problem [1]. CKD mortality almost doubled between 1990 and 2010, and by the end of 2013, over three million people were undergoing renal replacement therapy (RRT) worldwide, two and a half million were on hemodialysis or peritoneal dialysis, and close to 700,000 had received a kidney transplant. These numbers are predicted to continue to rise as the worldwide prevalence of CKD increases at a rate of 6% per year. Chronic kidney disease (CKD) is a national public health problem [2] that afflicts a broad cross-section of individuals in our society that has become recognized globally as an important cause of premature morbidity and mortality. However, it is particularly endemic among persons of historically recent African descent. In the United States, African Americans (AAs) are only 13% of the US population but are 32% of the CKD-dialysis population [3,6]. An earlier onset of hemodialysis initiation for CKD is correlated with genetic variation in the APOL1 gene [62].
Methods
To test our hypothesis that APOL1 allelic status is associated with CKD susceptibility, contributes to the current health disparity in kidney disease, and that the population frequency of the compound double heterozygote G1/G2 has been enhanced by recent microevolutionary events among Africans of the transatlantic Diaspora (i.e., Legacy African Americans), we consulted the existing scientific and historic literatures and, on the basis of these data, developed a model of the interactions of evolutionarily-important circumstances within their historical contexts and contemporary health disparities in a vulnerable, case-appropriate population. Data was solicited from the following sources:
- PubMed (https://pubmed.ncbi.nlm.nih.gov/),
- CDC Africa (http://africacdc.home; http://www.cdc.gov)
- WHO fact sheet on human African trypanosomiasis (https://www.who.int),
- Trypansomes Database (http://www.tanpaku.org),
- Trypanosome_brucei- Ensembl Genome 53 (https://protists.ensembl.org),
- Empires of West Africa Database (https://www.worldhistory.org),
- African History Sourcebook (https://sourcebook.forham.edu/Africa/africabook.asp),
- USRDS data (http://www.usrds.org, https://www.niddk.nih.gov),
- CKD-CDC
- (https://nccd.cdc.gov)(https://bmcnephrol.biomedcentral.com,https://www.kidney.org,
- https://www.frenova.com), and the
- Trans-Atlantic Slave Trade Database (https://www.slavevoyages.org/voyage/database).
No date limits were imposed upon the search for clinical and parasitological data; however, for the purpose of this study, our historical data was restricted to 1500 to 1900 CE. Our effort was to be comprehensive and inclusive, but temporally more recent references were given preference in our model construction and analysis. Our analysis did not include data from the Intra-American Slave Trade Database as these were not available at the time of our analyses.
Results
Of the numerous genes that have been associated with increased risk for chronic kidney disease, the APOL1 gene is among the most significant. The APOL1 gene codes for a high-density lipoprotein which binds to apolipoprotein A-I. Apolipoprotein A-I is an abundant plasma protein and is the major apoprotein of HDL. It forms most of the cholesteryl esters in plasma and promotes efflux of cholesterol from cells. This apolipoprotein L family member plays a role in lipid exchange and transport throughout the body, as well reversing the pattern of cholesterol transport from peripheral cells to the liver [4]. G1 and G2 are genetic variations in the APOL1 gene with G0 being the wild-type variant [5]. G1 is the major APOL1 risk allele for hypertension induced CKD in Central Africa, [56] a major site of source populations for Africans of the Atlantic Diaspora to the Americas. The APOL1 gene is a member of a family of six genes (APOL1, APOL2, APOL3, APOL4, APOL5 and APOL6), that are all located in close proximity on chromosome 22. Twenty-four different transcript variants encoding different isoforms have been found for this gene as of May 2023, representing coding sequence variants, synonymous variants, and missense variants (see 4). The APOL1 kidney risk variants are in the terminal exon of APOL1 within the SRA-binding domain on chromosome 22. (Table 1) summarizes the annotations for a few of the more common variants, indicating the linked mutational changes and associated phenotypes.
Table 1: Summary of specific major phenotypic effects in commonly encountered mutational changes in APOL1. Data accessed from [44-47]. Explanatory Box 1 provides more detail on the APOL1 variants associated with Trypanosoma brucei spp.
While an array of predisposing socioeconomic, political, genetic, and environmental conditions can initiate chronic kidney disease, APOL1-associated kidney disease is thought to account for a substantial proportion of CKD in AAs [7]. Population-based studies showed that APOL1 high-risk variant alleles were associated with an increased risk of CKD, [7] a 5.7-fold (95% CI 3.6–8.9) greater odds of incident albuminuria, [9] lower age of dialysis initiation, greater risk of incident hypertension, diabetes, but not acute kidney injury (AKI), hospitalizations, and mortality compared to European Americans [12]. The African American (AA) population has a total lifetime risk of ~0.8% (1 in 125) to develop focal segmental glomerulosclerosis (FSGS). A particular type of kidney lesion called focal segmental glomerulosclerosis (FSGS) causes a disease in which scar tissue develops on the glomeruli, The glomeruli are the small parts of the kidneys that filter waste from the blood. The G1 and G2 alleles of APOL1 increase this risk of scarification. The aggregate frequency of either of these two alleles in AAs is about 35% (1 in 3 individuals). For those persons with zero risk alleles, the risk of developing FSGS is 0.2%; for persons with 1 risk allele, it is 0.3%; and for persons with 2 risk alleles, it is 4.25% [13]. While it was known that the APOL1 genetic variants exert a protective action against African sleep sickness, the unexpected finding was that these, APOL1 variants also cause kidney disease [14]. It is not yet known if the risk for kidney disease is greater in the compound double heterozygote (Gi/G2) than in individuals with either the G1/G1 or G2/G2 phenotypes.
Genetics and epidemiology of APOL1 risk alleles in african americans and correlation with CKD
In genomics, a genome-wide association study (GWAS), us frequently used to link a set of genetic variants in different individuals with a disease condition. GWAS has revealed that a substantial fraction of the previously unexplained disparity in the incidence of end-stage renal disease between African Americans and European Americans can be explained by differences in the frequencies of the 2 alleles APOL1, G1 and G2. The APOL1 G1 allele consists of 2 missense mutational variants in very high linkage disequilibrium (APOL1 p. S342G, rs73885319[A;G]; APOL1 p.I384M, rs60910145[G:T]) or (rs71785313(-;TTATAA), rs71785313(D;I)), and the G2 allele is a 2–amino acid deletion (APOL1 p.delN388/Y389, rs60910145).
Although the effects of APOL1 risk variants on kidney disease in continental Africans is understudied (60), in admixed South Africans, it has been reported that, the frequency of the risk alleles of G1: rs73885319, G2: rs60910145 and G1:rs71785313 were respectively, 3.6%, 3.4%, and 5.8%, resulting in a 1.01% frequency of the APOL1 two-risk allele phenotypes (G1:G1 or G1:G2 or G2:G2). The presence of the two-risk alleles increased serum creatinine in South Africans with a corresponding reduction in eGFR (either MDRD or CKD-EPI based) [66]. In the United States, 30%−35% of AAs carry at least one APOL1 risk allele [61]. Between 13-14% of African Americans carry two APOL1 risk alleles, accounting for the high CKD burden in this population [32]. The compound double heterozygote genotype with alleles G1/G2 of the APOL1 gene, together increases the risk for kidney disease about 6-fold. The combination is considered pathogenic and of potential clinical significance both to the individual as well as for kidney transplantation considerations. 12-13% of African Americans carry the compound double heterozygote G1/G2 (31). Case control studies among African Americans with non-diabetic kidney disease showed that those individuals with two APOL1 high risk variants (G1/G1, G2/G2), or compound heterozygotes (G1/G2), are at a 10.5-fold (95% Confidence Intervals (CI) 6.0–18.4) greater risk of biopsy-proven focal segmental glomerulosclerosis (FSGS)-associated ESRD, 7.3-fold greater risk of hypertensive ESRD (95% CI 5.6–9.5), and 29-fold greater risk of HIV associated nephropathy (HIVAN) ESRD when compared to African Americans with only one or no copies of the APOL1 alleles. The risk of development of chronic kidney disease (CKD) is already 3–5 fold higher in the African American population. This increased risk is partly attributed to these two African ancestral genetic variants in the C-terminal domain of the APOL1 gene on chromosome 22 [33] and having the double heterozygote phenotype only amplifies this risk. African Americans carrying two APOL1 risk alleles have increased risk of myocardial infarction (odds ratio of 1.8) and mortality and having two APOL1 risk alleles increases the risk for peripheral vascular disease (determined non-invasively by ankle-brachial index) [34]. These compound double heterozygotes are at a 1.5- to 2.5-fold increased risk of chronic kidney disease [5]. Their chronic kidney disease risk appears to be triggered by either hypertension, type 2 diabetes, or HIV.
Background on the parasite
Trypanosomes are unicellular parasites that live in the blood plasma, the lymph and other tissues of vertebrate hosts including humans. These flagellate protozoans can cause a variety of diseases including fatal sleeping sickness. The trypanosomes cause serious economic losses and impose a severe public health burden. In West and Central Africa, the T. b. ambience parasite typically causes a chronic disease profile, while the zoonotic T. b. Rhodesians sub-species, located in Eastern and Southern Africa sylvatic reserves, results in a more rapidly progressing, acute infection. T. b. gambiense accounts for over 95% of infections [15]. In humans and other primates [16], APOL1 variants evolved as an innate resistance factor against trypanosomes and, possibly, other pathogens in Africa.
Figure 1: Depicts the relationship between the parasite, wild and domesticated ungulate species, and the evolutionary pressure on the APOL1 variants G1 and G2.
Figure 2: Shows the current geographical ranges for Trypanosoma brucei gambiense and Trypanosoma brucei rhodesiense.
Mechanisms of Apol1-mediated pathogen lysis
In Africa, trypanosomes are protozoan parasites that affect both humans and animals primarily living in economically poor rural areas. The diseases they cause are a major constraint on health and agricultural development. The APOL1 G1 (NM_001136540: c.1024A>G, p.Ser342Gly and NM_001136540:c.1152T>G, p.Ile384Met) and G2 (NM_001136540:c.1160_1165delATAATT) risk alleles render their carriers a natural resistance to infection by most African trypanosomes species because of a lytic protein component of APOL1. However, human-infective trypanosomes, T. b. Rhodesians found in more forested areas of East and South Africa, and T. b. Gambians in more populated, humid agricultural regions of West and Central Africa, have evolved separate mechanisms to respond to the gene’s lytic protein and cause disease in humans. Presumably, G1 originally protected against T.b.gambiense until the parasite evolved mechanisms to thwart the lytic protein of this APOL1 variant. T.b. rhodesiense, which maintains a sylvatic reservoir, remains susceptible to the lytic protein of APOL1 G2 [19-20]. Since the less common G2 variant can kill the T. b. Rhodesians parasites that are mostly found in East (21)(16) and South Africa, this suggests that the selective pressures observed in West and Central Africa for the T.b.gambiense subspecies of the flagella may not currently hold for the subspecies more common in East and South Africa. Geospatial oscillations in adaptation remind us of the evolutionary arms race influencing trypanosomiasis intensity and distribution relative to the APOL 1 alleles and that African sleeping sickness has been a positive selective agent for APOL1, albeit in different contexts within continental Africa.
How do the APOL1 alleles reduce the viability of the Trypanosoma flagella? In the human with either the G1 or G2 allele, the APOL1 gene product circulates in a specialized high-density lipoprotein (HDL), along with the haptoglobin-related protein, which acts as a receptor for entry into the trypanosome. The APOL1 trypanolytic activity is determined by 3 protein domains encoded by the terminal APOL1 exon: a pore-forming domain, a pH-sensitive membrane-addressing domain, and the serum resistance-associated protein (SRA)-interacting domain. The flagella are inactivated by APOL1 protein expression. These genetic variants acts by stopping the reproduction and spread of the parasite in the body. In this way, these genetic variants help their carriers avoid getting chronic trypanosomiasis. The APOL1-HDL particle also circulates connected to the IgM molecule [22], and these antibodies may serve as a back-up immunological anti-Trypanosoma response [23].
The protein expressed by these APOL1 genetic variants occurs in organs such as liver, pancreas, and kidney, as well as various types of cells, such as mononuclear phagocytes, placental cells, neurons in the prefrontal cortex and endothelial cells. In the kidney, APOL1 genetic variants were found to be expressed in endothelial cells, on the epithelium of the proximal convoluted tubule, podocytes, renal arteries, and renal arterioles. This finding may explain the pattern of clinical disease (e.g., glomerulosclerosis plus a component of vascular involvement and interstitial fibrosis) that we see within the compound double heterozygote G1/G2. APOL1-genetic variant associated pathologies have been observed in biopsies that, in addition to vascular and interstitial fibrosis, patients with the HRG have significantly more tubular atrophy [24].
Migration-influenced changes in admixture patterns
From 700 CE-1900 CE, the Millennia of Enslavement, Africans were enslaved opportunistically from diverse parts of the African continent over extended time periodsin a frenzy of competitive European and Arab demands for African labor in their colonies. From 1500 CE to 1900 C.E., the enslavement of 12.5 million Africans that were transported to the Americas, but many more were captured in Africa, disrupting historic empires and kingdoms and complex ecologically reinforced gene-environment interactions. The consequences of these disruptions still reverberate among descendant communities, including in their disproportionate burden in certain health disparities and continuous political instabilities. Numerous African empires and kingdoms were actively engaged in the warfare and social disruptions that led to the kidnapping, transport, sale, and transfer of Africans to the Americas. The trauma of this process was amplified by its multi-century duration and broad geospatial scope. For the first time, Africans who had not been in recent contact with each other, were brought together under the conditions of captivity. Over 16generations, reproductive unions occurred between individuals with ancestries from previously geographically distinct regions of continental Africa. These multi-generational interregional African-African admixture events altered previously existing directional selective pressures in specific indigenous groups, undermining local adaptations and creating new genomic combinations among the emerging African Americans. We know that there were different proportions of Africans from specific areas brought into North America (Table 2) and these proportional variations may have been reflected in the regional predominance of particular African affinities during the initial centuries of African residence in the Americas. Overtime and with extensive interregional African-African admixture, specific African continental genetic markers would have been diluted and homogenized. The subsequent migrations of African Americans within the Americas, e.g., The Great Migrationfrom approximately 1910 CE – 1970 CE [37] relocated six million African Americans from the American South to Northern, Midwestern, and Western US states, further diluting and homogenizing original genetic African distinctions.
Geographical Region of Africa |
Major African Export Sites for Kidnapped Africans |
Percentage of Enslaved Africans imported to Chesapeake Bay |
Percentage of Enslaved Africans Imported to Carolina Coast |
Percentage of Enslaved Africans Imported to Mississippi Delta |
Average Regional Totals of Imported Enslaved Africans into North America |
|
|
Associated current US States: MD, VA, DC, DE |
Associated current US States: NC, SC, GA |
Associated current US States: LA, MS, AL, AK |
|
WEST |
Senegambia |
15% |
23% |
29% |
22% |
|
Upper Guinea |
11% |
18% |
6% |
12% |
|
Gold Coast |
16% |
9% |
2% |
9% |
|
Bight of Benin |
Negligible |
3% |
25% |
9% |
|
Bight of Bonny |
38% |
Negligible |
8% |
15% |
CENTRAL |
Loango Coast |
16% |
40% |
28% |
15% |
SOUTH EAST |
Mozambique |
4% |
Negligible |
2% |
2% |
TOTALS |
|
100% |
100% |
100% |
|
Table 2: African Export Sites for North American Atlantic Diaspora populations. Intra African variability in forced migrations of enslaved Africans to North America.
While the significance of interregional African-African admixture has been largely ignored in the scientific literature, it remains the most important component of the genetic make-up of Africans of the trans Atlantic Diaspora. Continental Africa retains tremendous human genetic variability due to the long residence of humans on the continent, its pronounced ecological diversity, and the accelerated rate of evolution in this most tropical of continents (see 25). Additionally, Africa has a complex population history, large population base, and dramatic variation in climate, diet, and exposure to infectious diseases, which result in high levels of genetic and phenotypic variation in African populations [74]. During the transatlantic trade in enslaved Africans, vulnerable individuals were drawn from a large cross section of African populations (as reflected in Table 2) and thus the potential for interregional African-African admixture in their descendant generations remained notable and with potentially important evolutionary repercussions.
Considering African and African American mating patterns in the Americas during the nearly 300 years of American Slavery, these were characterized by the regular practice of intentional breeding and forced reproduction of enslaved Africans by the European and European-American owners of these individuals. The intention of this coercion was to increase the psychological subjugation and disorientation of the enslaved community by deliberately mixing Africans from different regions and ethnic groups to suppress the tendency to effectively rebel, destroy the opportunities for enslaved Africans to unify around families, promote detribalization, and to enhance the profits of slave owners by increasing the pool of enslaved individuals for labor without incurring the cost of the purchase of new forced immigrants. The emphasis on mandatory reproduction to increase the numbers of enslaved individuals was particularly important after 1807 when the transatlantic trade in enslaved Africans was stifled by the British and the domestic trade in enslaved persons became increasingly significant. In the 19thcentury during the last century of enslavement and just after emancipation, African American marriages were often not legally recognized, but were common [26]. The Civil Rights Act of 1866 defined the rights of freed people to own, sell, or lease personal and real property; enter contracts; and to be entitled to basic human rights. With the passage of the Thirteenth Amendment to the Constitution of the United States on December 6, 1865, African Americans could also marry freely.
As a byproduct of the trade in enslaved Africans, individuals from diverse ecological settings in Africa were brought together in the Americas. At this time, Africans from West and Central Africa, including carriers of the G1 variant of the APOL1 allele, encountered Africans from Southern and East Africa, including carriers of the G2 variant of the APOL1 allele. The demand for labor on behalf of the colonial European and Euro-American powers was so great in the Americas, that no region in which enslaved persons were kept experienced over population. African-African admixtures among Africans was the norm and sex-biased admixture between European males and African women was tolerated. Numerous studies have estimated the rate of European admixture in African Americans to be between 10-20% with important regional variation [27]. Genome-wide ancestry estimates of African Americans show average proportions of 73.2% African, 24.0% European, and 0.8% Native American ancestry [28]. Less well documented are the predominant interregional (i.e., intra-African) African-African admixtures that occurred in the Americas, that brought diverse carriers of distinct genetic adaptations and with divergent prior evolutionary histories (with Trypanosoma subspecies and other pathogens) together under the disruptive conditions of color-based enslavement, institutionalized racial discrimination, and segregation. In the Americas, the detribalization of Africans overtime facilitated by the gradual diminution of cultural continuity likely permitted the intermarriages of West and Central Africans with East and South Africans, increasing the probability of generating the complex double heterozygote G1/G2 in their offspring. The high level of kidney disease in African Americans reflects an assortment of accumulated socioeconomic and legal-political disadvantages but is also a consequence of the robust interregional African-African genetic admixtures initiated at least 16 generations ago. Today, the admixtures in African Americans reflect ancestries from many different African populations, but because of the limitations in current genomic reference sample databases for continental Africa, our annotation of the distinctions of these African ancestries are restricted. The African origins of the APOL1 genetic variants and their connection with CKD still generate many unanswered questions.
The African origins of the APOL1 genetic variants and their connection with CKD
While there remains a paucity of genomic studies in Africa, there are some studies that seem to reinforce our working hypothesis. In the Democratic Republic of the Congo, children with APOL1 risk alleles demonstrate early kidney damage [64], and this region of Central West Africa was a major ancestral geographical source for AAs. Additional studies confirming the African origins for these APOL1 variants comes from a second study of children in the Democratic Republic of the Congo [71] where clinical and genetic factors were associated with kidney complications in pediatric patients with sickle cell anemia. Additional studies demonstrating the CKD-associated APOL1 variants as being of African origin come from Cameroon [70] and South Africa [72].
Proposed mechanisms of APOL1 interactions in CKD
How do the protein products of the APOL1 genetic variants interact with the kidney? We know that the APOL1 C-terminal variants G1 and G2 are linked to CKD epidemiologically. Expression of these variants result in the impairment of the blood filtration activity of the kidney, possibly through disturbed endothelial function (36). A characteristic of the disease associated with having both G1 and G2 APOL1-genetic variants is the strong association of glomerular pathology with the type I IFN inflammatory response, such as occurs with viral infection. APOL 1 G1- or G2-mediated inhibition of APOL 3 best explains the resulting chronic kidney disease. Thus, kidney disease is not only genetically linked to APOL 1 G1 and G2, but also to natural APOL3 knockout (13). In vitro, APOL3 knockout increases endothelial permeability, reduces wound repair and limits tubule formation [36], and these mechanisms may also be replicated in vivo. Indeed, presence of the double complex heterozygote G1/G2 results in an increased odds of early kidney disease, and infection in carrier the Democratic Republic of the Congo [39].
As previously mentioned, the APOL3 gene is located on chromosome 22 and the encoded protein is found in the cytoplasm, where it seems to affect the movement of lipids, allows the binding of lipids to organelles, and possibly be involved in inflammatory processes and cell death mechanisms. Other mechanisms which have been proposed are that these variants may create pores in the kidney cell membrane or cause mitochondrial damage [52-53], perhaps through lipid dysregulation [58-59].
There have also been studies in continental Africa and in the Americas that have looked at the APOL1-CKD association in sickle cell trait. APOL1 has been associated with sickle cell nephropathy. This illustrates the interaction of two genetic disorders more commonly seen in peoples of African descent further interacting to increase the risk for kidney disease, This interaction places African Americans with sickle cell disease at an even higher risk for CKD [54]. APOL1 genetic variants may have a significant role in kidney transplantation. APOL1 may not just increase the risk for transplant graft failure in the recipient of an APOL1 high risk variant kidney but may increase the risk of End Stage Renal Disease (ESRD) in the living donor. There are studies that suggest that kidney donors with high-risk variants may have a higher risk for kidney failure after donation (69). Population studies have also shown that AA who donate kidneys may have a much higher risk for the future development of ESRD and it makes sense to postulate that this may be explained, in part, by APOL1 genetic variants [55].
Discussion
A previously discussed, the human APOL family is a cluster of six genes that have evolved rapidly under positive selection in primates. This means that these genes are under adaptive selection and must have some beneficial effects. However, aside from the founding member APOL1the functions of the intracellular APOL family members are not fully understood (40). APOL1 and chronic kidney disease interactions appear to be mediated through APOL3 resulting in the disruption of the vasculature of the intact kidney. It is interesting how the APOL1 alleles G1 and G2 maintain anti-trypanosomal lysis abilities and have undergone positive selection in Trypanosoma-infested environments. The distribution of these APOL1 genetic variants closely follows the geospatial distribution and population’s historic exposure to specific subspecies of the Trypanosomal parasite. As such, these human genetic traits, and the protozoan flagella to which they respond can be thought of as being in a reciprocal coevolutionary entanglement where the frequency of one influence the viability of the other.
In West and Central Africa where T.b.gambiense predominates, G1 no longer appears to confer the definitive selective advantage it may have had 16 generations ago. The parasite has evolved, facilitated, in part, by regular contact with humans via their agricultural practices. T.b.rhodesiensis, found in East and South Africa, retains its vulnerability to the lytic capabilities of G2. In North America however, these two subspecies of Trypanosoma are not found. In their absence, the same adaptive human genetic traits that evolved under positive selective pressures have become maladaptive and contribute to a higher incidence of chronic kidney disease in a vulnerable population. This is a cogent example of a gene-environment mismatch and a counterproductive interaction of evolution and health disparities: a previously adaptive genetic trait in a new environment initiates an unusual (and unexpected) pattern of maladaptation.
The forced transport of Africans from diverse geographical regions and ecological zones of continental Africa to the Americas and mandated African-African admixtures set the stage for the greatly enhanced gene flow between Africans from different, and previously non-interactive areas of Africa. A pattern of regional adaptations that was in existence for generations was disrupted by their enslavement and transatlantic transport and new patterns of gene flow in the Americas ushered in the increased potential to produce the double complex heterozygote G1/G2. This elevated presence of two risk factors in kidney disease contribute to the current health disparity in end stage renal disease. While G1/G1 homozygotes and G2/G2 homozygotes suffer an increased risk for kidney disease, the presence of complex heterozygotes amplifies the CKD risk. G1 and G2 retain lytic abilities even in the absence of Trypanosomes or other lysable units. G1/G2 disrupts other systems, including APOL3. APOL3 is a potent effector protein capable of a detergent-like action, extracting lipids from membranes of bacteria in the cytosol, killing cytosol-invasive bacteria (such as Listeria monocytogenes, Shigella flex Neri, Burkholder a pseudo mallei, B. thalialandensis, Francis Ella tularensis and Rickettsia spp. Reducing podocyte dysfunction could involve knocking out APOL3, but because of APOL3’s antibiotic properties, this intervention would presumably increase phenotypic susceptibility to these cytosol-invasive bacteria.
Recently, the New York Times published an article [50] on the health disparities surrounding chronic kidney disease and its prevalence in African Americans. As biotech companies begin conducting research on the APOL1 gene's contribution to CKD, the article raised concerns about a subsequent disregard for the impact of economic and social barriers in affecting the health of Legacy African Americans. Research on both the environmental and genetic causes of CKD is imperative. A related article [51] illustrates another way in which the APOL1 genetic variants affect Legacy African Americans. Because of the well-known prevalence of CKD in this population, kidneys from deceased African American donors are automatically considered a higher risk for transplant. However, not every African American has the APOL1 alleles which increase their risk of developing CKD. With widespread genetic screening and further research on the effect of the APOL1 gene on CKD, transplant organs would be considered for one's genome rather than their skin color.
Building upon the interactive model provided in this paper, future research should be aimed at further clarification of the gene-gene and gene-environment interactions in APOL1 and CKD. Preemptive clinical testing of APOL1 status [57] would be particularly helpful for the African-descended community to identify the presence of risk alleles, intercept then development of environmental triggers for CKD, and reduce CKD mortality. APOL1 variants are found throughout the Atlantic African Diaspora as well as among Africans living in Europe [73]. We need an improved characterization of various APOL1 genetic variants and phenotypes particularly among these African-descended peoples, and a deeper understanding of the networks mediating of APOL1-associated pathways resulting in cellular injury and dysfunction in the kidney (and other) cells. Finally, we must develop novel interventions therapies based upon the theoretical premises of evolutionary medicine. The interactions discussed in this paper can provide a foundation for CRISPR Cas9 and other genetic engineering interventions that reduce the pathology to the kidney vasculature associated with the G1/G2 double heterozygote without diminishing the adaptive lytic capabilities of these proteins. Our goal in this paper has been to explore the gene-gene and gene-environment interactions in APOL1 and chronic kidney disease and to demonstrate in this case study how human microevolution, when historically contextualized, can impact current health disparities.
Acknowledgements
This comprehensive review is dedicated to Dr. Clive Callender, professor of surgery and preeminent kidney transplant surgeon at Howard University College of Medicine. Dr. Callender founded the National Minority Organ Tissue Transplant Education Program (MOTTEP), more than tripling African American organ donation rates. Dr. Callender has been a leader in the fight against discriminatory policies in organ allocation and an advocate of organ donation and transplantation, particularly among African Americans who disproportionately experience chronic kidney disease.
We thank the All of Us Researcher Academy (NIH) and RTI for their support through the conduct of this research and the write-up of this interdisciplinary paper. Members of the QuadGrid Human Analytics Research Team (part of QuadGrid Data Lab) who also contributed to this paper include Obinna S. Asawabelem, Kaitlin Kenton, Jade Telesford, and Shihyun Kim.
Declaration
Ethics approval and consent to participate All data generated for this database were accessed from historical materials. No living populations of humans or animals were used in the construction of these materials.
Consent for publication
All authors of this paper agree to its submission for publication consideration.
Availability of data and materials
The majority of databases consulted in this paper are already in the public domain. Data from the AEK database will be made available to the public upon completion of the project.
Competing interests
The authors declare no competing interests.
Funding
No external funding was received for the production or analysis of the data reported on in this paper but our efforts were under the umbrella of support from All of Us Researchers Academy. This work is supported by the Division of Engagement and Outreach, All of Us Research Program, National Institutes of Health under award number 1OT20D028395-01.
Author’s Contributions
The project was conceived by FJ, technically supported by HJ, the database and its analyses were developed by RF, SS, NG, VM along with FJ and HJ. Original graphics were developed by NG, VM, and FJ. Bibliographic assistance was provided by RF and SS.
References
-
Bikbov B, Purcell CA, Levey AS, Smith M, Abdoli A, et al. (2020) GBD Chronic Kidney Disease Collaboration. Global, regional, and national burden of chronickidney disease, 1990-2017: a systematic analysis for the Global Burden of Disease Study 2017. The Lancet. 395(10225):709-33.
-
Nicholas SB, Kalantar-Zadeh K, Norris KC. (2015) Socioeconomic disparities in chronic kidney disease.Adv chronic kidney Dis. 22(1):6–15.
-
Friedman DJ, Pollak MR. (2020) APPOL1 and kidney disease from genetics to biology. Ann Rev Physiol. 82:323-42.
-
Danesh pajouhnejad P, Kopp JB, Winkler CA, Rosenberg AZ. (2022) The evolving story of apolipoprotein L1 nephropathy: the end of the beginning. Nat Rev Nephrol. 18(5):307-20.
-
Siemens TA, Riella MC, Moraes TP, Riella CV. ( APOL1 risk variants and kidney disease: what we know so far. J Bras Nefrol. 40(4):388–402.
-
Alexander GB, Akwo E, Robinson-Cohen C, Lee K, Lynch J, et al. (2019) Association of APOL1 Risk Alleles with Cardiovascular. Disease in Blacks in the Million Veteran Program. Circulation. 140(12):1031–40
-
Umeukeje EM, Young BA. (2019) Genetics and ESKD Disparities in African Americans. Am J kidney Dis. 74(6):811–21.
-
Foster MC, Coresh J, Fornage M, Astor BC, Grams M, et al. (2013) APOL1 variants associate with increased risk of CKD among African Americans. J Am Soc Nephrol. 24(9):1484–91.
-
Peralta CA, Bibbins-Domingo K, Vittinghoff E, Lin F, Fornage M, et al. (2016) APOL1 Genotype and Race Differences inIncident Albuminuria and Renal Function Decline. J Am Soc Nephrol. 27(3):887–93.
-
Kanji Z, Powe CE, Wenger JB, Huang C, Ankers E, et al. (2011) Genetic variation in APOL1 associates with younger age athemodialysis initiation. J Am Soc Nephrol. 22(11):2091–97.
-
Tzur S, Rosset S, Skorecki K, Wasser WG. (2012) APOL1 allelic variants are associated with lower age of dialysis initiation and thereby increased dialysis vintage in African and Hispanic Americans with non-diabetic end-stage kidney disease. Nephrol Dial Transplant. 27(4):1498–1505.
-
Reeves-Daniel AM, DePalma JA, Bleyer AJ, et al. The APOL1 gene and allograft survival after kidney transplantation. Am J transplant. 11(5):1025–30.
-
Kopp JB, Nelson GW, Sampath K, Johnson RC, Genovese G, et al. (2011) APOL1 genetic variants in focal segmental glomerulosclerosis and HIV-associated nephropathy. J Am Soc Nephrol. 22(11):2129-37.
-
Pays E. (2020) The Mechanism of Kidney Disease due to APOL1 Risk Variants. J Am Soc Nephrol. 31(11):2502-05.
-
Kennedy PGE, Rogers J. (2019) Clinical and Neuropath genetic Aspects of Human AfricanTrypanosomiasis. Front Immunol. 10:39.
-
Cooper A, Capewell P, Clucas C, Veitch N, Weir W, et al. (2016) A Primate APOL1 Variant That Kills Trypanosoma brucei gambiense. PLoS Negl Trop Dis. 10(8):e0004903.
-
Thomson R, Genovese G, Canon C, Kovacics D, Higgins MK, et al. (2014) Evolution of the primate trypanolytic factor APOL1. Proc Natl Acad Sci U S A. 111 (20) E2130-139.
-
Kimuda MP, Noyes H, Mulindwa J, Enyaru J, Alibu VP, et al. (2018) No evidence for association between APOL1 kidney disease risk alleles and Human African Trypanosomiasis in two Ugandan populations. Plos Negl Trop Dis. 12(2):e0006300.
-
Masimango MI, Jadaul M, Binns-Roemer EA, Davis VA, Sumaili EK, et al. (2022) APOL1 renal risk variants and sickle cell trait association with reduced kidney function in a large Congolese population-based study. Kidney Int Rep. 7(3):474-82.
-
Kruzel-Davila E, Skorecki K. (2017) The double-edged sword of evolution. ELife. 6:e29056.
-
Cooper A, Ilboudo H, Alibu VP, Ravel S, Enyaru J, et al. (2017) APOL1 renal risk variants have contrasting resistance and susceptibility associations with African trypanosomiasis. ELife. 6:e25461.
-
Genovese G, Friedman DJ, Ross MD, Lecordier L, Uzureau P,et al. (2010) Association of trypanolytic ApoL1 variants with kidney disease in African Americans. Science. 329(5993):841-5.
-
Kozlitina J, Zhou H, Brown PN, Rohm RJ, Pan Y, et al. (2016) Plasma Levels of Risk-Variant APOL1 Do Not Associate with Renal Disease in a Population-Based Cohort. J Am Soc Nephrol. 27(10):3204–19.
-
Magez S, Schwegmann A, Atkinson R, Claes F, Drennan M, et al. (2008) The role of B-cells and IgM antibodies in parasitemia, anemia, and VSG switching in Trypanosoma brucei-infected mice. PLoS Pathog. 4(8):e1000122.
-
Schelling VR. (2015) Tubular atrophy in the pathogenesis of chronic kidney disease preogression. Pediatr Nephrol. 31(5):693-706
-
https://people.wku.edu/charles.smith/biogeog/DOBZ1950.htm
-
https://www.hup.harvard.edu/catalog.php?isbn=9780674237452
-
Zakharia F, Basu A, Absher D, Assimes TL, Go AS, et al. (2009) Characterizing the admixed African ancestry of AfricanAmericans. Genome Biol. 10(12):R141.
-
Bryc K, Durand EY, Macpherson JM, Reich D, Mountain JL. (2015) The genetic ancestry of African Americans, Latinos, and European Americans across the United States. Am J Hum Genet. 96(1):37–53.
-
https://escholarship.org/content/qt8cn4z1st/qt8cn4z1st_noSplash_558f033474213438f7d7c53238338659.pdf?t=np7zvf
-
Ito K, Bick AG, Flannick J, Friedman DJ, Genovese G, et al. (2014) Increased burden of cardiovascular disease in carriers ofAPOL1 genetic variants. Circulation research. 114(5):845–50.
-
Friedman DJ, Kozlitina J, Genovese G, Jog P, Pollak MR. (2011) Population-based risk assessment ofAPOL1 on renal disease. J Am Soc Nephrol. 22(11):2098–2105.
-
Wasser WG, Tzur S, Wolday D, Adu D, Baumstein D, et al. (2012) Population genetics of chronic kidney disease: the evolving story of APOL1. J Nephrol. 25(5):603-18.
-
Reiner AP, Susztak K. (2016) APOL1 Variants: From Parasites to Kidney Function to Cardiovascular Disease. Arterioscler Thromb Vasc Biol. 36(2):219–20.
-
Limou S, Nelson GW, Kopp JB, Winkler CA. (2014) APOL1 kidney risk alleles: population genetics and disease associations. Adv Chronic Kidney Dis. 21(5):426–33.
-
Khalil A, Poelvoorde P, Fayyad-Kazan M, Rousseau A, Nuyens V, et al. (2018) Apoliporotein L3 interferes with endothelial tube formation via regulation of ERK1/2, FAK and Akt signaling pathway. Atherosclerosis. 279:73-87.
-
Tolnay SE. (2003) The African American “Great Migration” and beyond. Ann Rev Soc. 29(2003):209-32.
-
Ekulu PM, Nkoy AB, Adebayo OC, Kazadi OK, Aloni MN, et al. (2021) A focus on the association of Apol1 with kidney diseasein children. Pediatr Nephrol. 36(4):777-88.
-
Gaudet RG, Zhu S, Halder A, Kim BH, Bradfield CJ, et al. (2021) A human apolipoprotein L with detergent-likeactivity kills intracellular pathogens. Science. 373(6552):eabf8113.
-
Harvest CK, Mail EA Autophagy May Allow a Cell to Forbear Pyroptosis When Confronted WithCytosol-Invasive Bacteria. Front Immunol. 13:871190.
-
Freedman BI, Kopp JB, Sampson MG, Susztak K. (2021) APOL1 at 10 years: progress and next steps. Kidney Int. 99(6):1296-1302.
-
Simarro PP, Cecchi G, Franco JR, Paone M, Diarra A, et al. (2012) Estimating and Mapping the Population at Risk of Sleeping Sickness. PLOS Negl Trop Dis. 6(10):e1859.
-
Li Q, Fan P, Bai H, Liu R, Huang Y, et al. (2009) Distribution and effect of apoL-I genotype on plasma lipid and apolipoprotein levels in Chinese normalipidemic and endogenous hypertriglyceridemic subjects. Clin Chim Acta. 403(1-2):152-5.
-
Genovese G, Friedman DJ, Ross MD, Lecordier L, Uzureau P, et al. (2010) Association of trypanolytic ApoL1 variants with kidneydisease in African Americans. Science. 329(5993):841-5.
-
McCarthy HJ, Bierzynska A, Wherlock M, Ognjanovic M, Kerecuk L, et al. (2013) Simultaneous sequencing of 24 genesassociated with steroid-resistant nephrotic syndrome. Clin J Am Soc Nephrol. 8(4):637-48.
-
Cohen DM, Mittalhenkle A, Scott DL, Young CJ, Norman DJ. (2011) African American living-kidney donors should be screened for APOL1 risk alleles. Transplantation. 92(7):722-5.
-
Jackson F. (2004) Human genetic variation and health: Ethnogenetic layering as a way of detecting relevant population substructuring. Br Med Bull. 69:215-35.
-
Jackson H, Jackson F. (2017) Geospatial distribution and population substructure of subgroups of US ethnic minorities: implications for perpetuation of health disparities and paucity of precision medicine. J Family Stren. 17(1):1-19.
-
https://www.nytimes.com/2022/05/17/health/kidney-disease-black-americans.html
-
www.nytimes.com/2022/05/17/health/kidney-transplants-blackamericans.html
-
Friedman DJ, Pollak MR. (2021) APOL1 Nephropathy: From Genetics to Clinical Applications. Clin J Am Soc Nephrol. 16(2):294-303.
-
Freedman BI, Limou S, Ma L, Kopp JB. (2018) APOL1-Associated Nephropathy: A Key Contributor to Racial Disparities in CKD. Am J Kidney Dis. 72(5 Suppl 1):S8-S16.
-
Zahr RS, Rampersaud E, Kang G, Weiss MJ, Wu G, et al. (2019) Children with sickle cell anemia and APOL1 genetic variants develop albuminuria early in life.Haematologica. 104(9):e385-e387.
-
Muzaale AD, Massie AB, Wang MC, Montgomery RA, McBride MA, et al. (2014) Risk of end-stage renal disease following live kidney donation. JAMA. 311(6):579-86.
-
Sumaili EK, Shemer R, Kruzel-Davila E, Cohen EP, Mutantu PN, et al. (2018) G1 is the major APOL1 risk allele for hypertension-attributed nephropathy in Central Africa. Clin Kidney J. 12(2):188-95.
-
Young BA, Fullerton SM, Wilson JG, Cavanaugh K, Blacksher E, et al. (2017) Clinical Genetic Testing for APOL1: Are we There Yet? Semin Nephrol. 37(6):552-57.
-
Ge M, Molina J, Ducasa GM, Mallela SK, Varona Santos J, et al. (2021) APOL1 risk variants affect podocyte lipid homeostasis and energy production in focal segmental glomerulosclerosis. Hum Mol Genet. 30(3-4):182-97.
-
Valsecchi M, Cazzetta V, Oriolo F, Lan X, Piazza R, et al. (2020) APOL1 polymorphism modulates sphingolipid profile of human podocytes. Glycoconj J.37(6):729-44.
-
Brandenburg JT, Govender MA, Winkler CA, Boua PR, Agongo G, et al. (2022) Apolipoprotein L1 High-Risk Genotypes and Albuminuria in Sub-Saharan African Populations. Clin J Am Soc Nephrol. 17(6):798-808.
-
Friedman DJ, Kozlitina J, Genovese G, Jog P, Pollak MR. (2011) Population-based risk assessment of APOL1 on renal disease. J Am Soc Nephrol. 22(11):2098-105.
-
Friedman D, Genovese G, Pollak MR, Thadhani R. (2011) Genetic variation in APOL1 associates with younger age at hemodialysis initiation. J Am Soc Nephrol. 22(11):2091-7.
-
AN P, Sezgin E, Kirk GD, Duggal P, Binns-Roemer E, et al. (2021) APOL1 variant alleles associate with reduced risk for opportunistic infections in HIV infection. Commun Biol. 4(1):284.
-
Ekulu PM, Nkoy AB, Betukumesu DK, Aloni MN, Makulo JRR, et al. (2019) APOL1 Risk Genotypes Are Associated with Early Kidney Damage in Children in Sub-Saharan Africa. Kidney Int Rep. 4(7):930-38.
-
Duran CE, Ramírez A, Posada JG, Schweineberg J, Mesa L, et al. (2019) Prevalence of APOL1 Risk Variants in Afro-Descendant Patients with Chronic Kidney Disease in a Latin American Country. Int J Nephrol. 2019:7076326.
-
Matsha TE, Kengne AP, Masconi KL, Yako YY, Erasmus RT. (2015) APOL1 genetic variants, chronic kidney diseases and hypertension in mixed ancestry South Africans. BMC Genet.002026:16-69.
-
Servais A, Gribouval O, Gaillard F, Antignac C. (2019) APOL1 risk genotype in Europe: Data in patients with focal segmental glomerulosclerosis and after renal transplantation. Nephrol Ther. 15 Suppl 1:S85-S89.
-
Langefeld CD, Comeau ME, Ng MCY, Guan M, Dimitrov L, et al. (2018) Genome-wide association studies suggest that APOL1-environment interactions more likely trigger kidney disease in African Americans with nondiabetic nephropathy than strong APOL1-second gene interactions. Kidney Int. 94(3):599-607.
-
Chang JH, Husain SA, Santoriello D, Stokes MB, Miles CD,et al. (2019) Donor' s APOL1 Risk Genotype and & quot; Second Hits & quot; Associated With De Novo Collapsing Glomerulopathy in Deceased Donor Kidney Transplant Recipients: A Report of 5 Cases. Am J Kidney Dis. 73(1):134-39.
-
Geard A, Pule GD, ChetchaChemegni B, Ngo Bitoungui VJ, Kengne AP, et al. (2017) Clinical and genetic predictors of renal dysfunctions in sickle cell anaemia in Cameroon. Br J Haematol. 178(4):629-39.
-
Adebayo OC, Betukumesu DK, Nkoy AB, Adesoji OM, Ekulu PM, et al. (2022) Clinical and genetic factors are associated with kidney complications in Africanchildren with sickle cell anaemia. Br J Haematol. 196(1):204-14.
-
Hassan MO, Duarte R, Dickens C, Dix-Peek T, Naidoo S, et al. (2020) APOL1 Genetic Variants Are Associated with Serum-Oxidized Low-Density Lipoprotein Levels and Subclinical Atherosclerosis in South African CKD Patients. Nephron. 144(7):331-40.
-
Hung RKY, Binns-Roemer E, Booth JW, Hilton R, Harber M, et al. (2022) Genetic Variants of APOL1 Are Major Determinants of Kidney Failure in People of African Ancestry With HIV. Kidney Int Rep. 7(4):786-96.
-
Egbuna O, Zimmerman B, Manos G, Fortier A, Chirieac MC, et al. (2023) Inaxaplin for proteinuric kidney disease in persons with two APOL1 variants. N Engl J Med. 388(11), 969-79.
-
https://the-cynosura.tumblr.com/post/24776417352/a-false-coloured-scanning-electron-microscope. Cynosura.
-
https://www.pinterest.com/pin/367817494536350482/ripomastigotas de Trypanosoma brucei (gambiense/ rhodesiense) en la sangre, protista causador de la Tripanosomiasis Africana. Ciencias.
-
https://www.notesonzoology.com/practical-zoology/classification-of-protozoa-4-types/2745.
-
https://healthtian.com/african-sleeping-sickness-african-trypanosomiasis/
-
Bannister A NHPA/Encyclopedia Britannica, Inc. https://www.britannica.com/animal/tsetse-fly/images videos. Accessed May 16, 2023
-
Linderman MD, Nielsen DE, Green RC. (2016) Personal Genome Sequencing in Ostensibly Healthy Individuals and the PeopleSeq Consortium. J Pers Med. 6(2):14.
-
Chen S, Francioli LC, Goodrich JK, Collins RL, Wang Q, et al. (2022) A genome-wide mutational constraint map quantified from variation in 76,156 human genomes. BioRxiv. 1-42.
-
https://www.pvamu.edu/tiphc/research-projects/the-diaspora-coming-to-texas/the-transatlantic-slave-trade-and-origins-of-the-african-diaspora-in-texas/#:~:text=The%20African%20Diaspora%20in%20what,as%20he%20is%20commonly%20known.
-
https://www.pvamu.edu/tiphc/research-projects/the-diaspora-coming-to-texas/the-transatlantic-slave trade-and-origins-of-the-african-diaspora-in-texas/.
-
Obikili N. 2015 The trans-Atlantic slave trade and local political fragmentation in Africa. 2015. AEHN. 69(4):1157-77.
-
http://www.amazon.com/Africa-Peoples-their-Culture-History/dp/0070440522.
-
Jackson H. (2023) FLC no date African Empires and Kingdoms Database (AEKD), Quad Grid Data Lab, https://quadgrid.com/.