Neuroprotective Effects of Neurotrophic Factors in Neurodegenerative Diseases: A Systematic Review
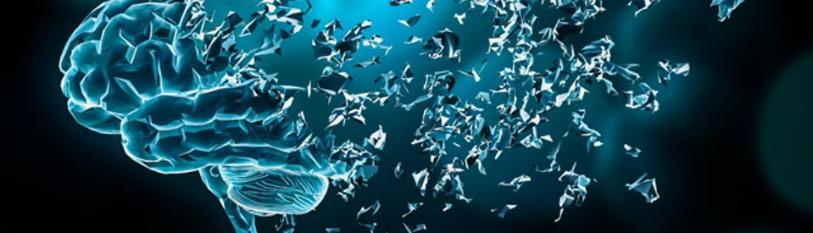
Rizwana Syed1*, Niharika Reddy2, Sarang Tusharbhai Mehta3, Amir Rasheed4 and Binay Kumar Paniyar5
1Apollo Institute of Medical Sciences and Research, Chittoor, India
2Department of Neuro-oncology, University of Kentucky, Lexington, USA
3Smt. N.H.L Municipal Medical College, India
4Nawaz Sharif Medical College Gujrat, Pakistan
5Harvard Medical School, USA
*Corresponding author: Rizwana Syed, Apollo Institute of Medical Sciences and Research, Chittoor, India.
Citation: Syed R. Reddy N, Mehta ST, Rasheed A, Paniyar BK. Neuroprotective Effects of Neurotrophic Factors in Neurodegenerative Diseases: A Systematic Review. J Neurol Sci Res. 4(2):1-13.
Received: June 04, 2024 | Published: July 18, 2024
Copyright©️ 2024 genesis pub by Syed R, et al.CC BY-NC-ND 4.0 DEED. This is an open-access article distributed under the terms of the Creative Commons Attribution-Non-Commercial-No Derivatives 4.0 International License. This allows others distribute, remix, tweak, and build upon the work, even commercially, as long as they credit the authors for the original creation.
Abstract
Neurodegenerative diseases (ND) are a significant global health challenge, characterized by theprogressive loss of neuronal structure and function. Current therapeutic approaches do not effectively target the underlying mechanisms of these diseases and often provide limited benefits. Neurotrophic factors have shown promise in promoting neuronal growth, differentiation, and survival, making them potential candidates for neuroprotection.
Keywords
Neuroprotective; Neurodegenerative diseases; Nervous system; Neurotrophic factor
Introduction
Neurodegenerative diseases (ND) are a significant global health challenge, characterized by the progressive loss of neuronal structure and function. Current therapeutic approaches do not effectively target the underlying mechanisms of these diseases and often provide limited benefits. Neurotrophic factors have shown promise in promoting neuronal growth, differentiation, and survival, making them potential candidates for neuroprotection. This systematic review aims to critically assess the existing literature on the neuroprotective effects of neurotrophic factors in various neurodegenerative diseases. We conducted an extensive search across electronic databases to identify relevant studies. After thorough data extraction and quality evaluation, we included studies that investigated the impact of neurotrophic factors on neurodegeneration. The included studies covered a range of neurodegenerative diseases, such as Parkinson's disease, Alzheimer's disease, Huntington's disease, and amyotrophic lateral sclerosis.
We analyzed 213 articles from reputable journals that were published between 2013 and August 10, 2023. We specifically focused on ten selected papers for a detailed examination. The results of our review further support the effectiveness of neurotrophic factors in mitigating neurodegeneration. Numerous neurotrophic factors have demonstrated the capacity to enhance neuronal function and support survival in various disease models. These factors include ciliary neurotrophic factor (CNTF), brain-derived neurotrophic factor (BDNF), glial cell line-derived neurotrophic factor (GDNF), and nerve growth factor (NGF). These effects are achieved through mechanisms such as reducing inflammation, promoting the growth of neurites, and modulating synaptic plasticity. It is important to note that neurotrophic factors utilize multiple signaling pathways and interact with specific pathologies associated with the diseases. However, challenges related to the stability, delivery, and potential side effects of neurotrophic factors are also discussed in the review. This emphasizes the necessity of developing targeted delivery systems to optimize the therapeutic potential of these factors.
While the majority of studies indicate positive effects of neurotrophic factors, there are some limitations in the literature. This systematic review offers a comprehensive assessment of the current research on the neuroprotective effects of neurotrophic factors in neurodegenerative diseases. Despite the challenges and constraints, the overall evidence suggests that neurotrophic factors have potential as therapeutic agents for these debilitating disorders. Further investigation is needed to understand the intricate mechanisms behind their effects and to develop strategies that enhance their clinical applicability. Ultimately, a deeper understanding of the interaction between neurotrophic factor signaling and neurodegeneration could pave the way for groundbreaking and targeted interventions, bringing hope to patients with neurodegenerative.
Our nervous system is a medium that makes us aware of our surroundings, provides us with the required stimulation to explore, and creates memories of all good and bad experiences that help us improve every day. Humans are sometimes rendered incapable of performing all that due to time-dependent consequences and genetic makeup, which causes suffering. We refer to them as neurodegenerative diseases (NDs) because the cause of them is degeneration [1, 2, 3]. Although neurons are not immortal, the progressive loss of neurons, neuron structure, and/or their functions, known as neurodegeneration, is central to the pathophysiology of several brain disorders [4] and is also a major health concern. Diseases with neurodegeneration as their hallmark feature are collectively termed NDs [5, 6]. The most common NDs include Alzheimer’s disease, Parkinson’s disease, prion disease, amyotrophic lateral sclerosis, motor neuron disease, Huntington’s disease, spinal muscular atrophy, and spinocerebellar ataxia [5, 7, 8, 9]. Neurodegeneration has been identified as the pivotal pathophysiological change in most brain-related disorders [10]. Regardless of the incessant efforts of modern science to develop a medical or surgical solution, the outcome has not been favorable. Neurodegenerative disorders (NDs) such as Alzheimer’s and dementia continue to be a clinical concern for most older people [11, 12].
Neurotrophic factors are those molecules that help a group of cells to survive. These polypeptides play central roles in nervous system development, from naturally occurring cell death to differentiation and neurite outgrowth. The acknowledged prototypical example and best-characterized neurotrophic factor is nerve growth factor (NGF), a 3-fold-structured serine proteinase that helps sympathetic and sensory neurons in the peripheral nervous system and also functions in the development and maintenance of cholinergic neurons [13]. BDNF stands as a distinguished member of these neurotrophins, contributing indispensably to neuronal survival, orchestrating axonal guidance, and sculpting the intricate landscape of activity-dependent synaptic plasticity [14].
All known neurotrophic factors are classified into these three families based on structural and functional similarity (Figure 1).
Figure 1: Brain Derived Growth Factor (BDNF), Nerve Growth factor (NGF), Neurotrophin-3 (NT-3), Neurotrophin-4 (NT-4), and Neurotrophin-6 (NT-6), Neurturin (NRTN), Glial Cell-derived Neurotrophin Factor (GDNF), Persphin (PSPN), Artemin (ARTN), Ciliary Neurotrophic Factor (CNTF), Leukemia Inhibitory Factor (LIF), Interleukin-6(IL-6), Interleukin-11(IL-11), Cardiotrophin-1 (CT-1), Cardiotrophin-Like Cytokine (CLC), and Oncostain-M (OSM).
Nerve growth factor (NGF) family: The nerve growth factor family consists of NGF, BDNF, neurotrophin-3 (NT-3), neurotrophin-4/5 (NT-4), and neurotrophin-6 (NT-6). Target-derived substances called neurotrophins work by preventing apoptosis in target-supporting neurons to increase their survival. NGF serves as both the model for the neurotrophin family and the first member of the NTF family to be discovered. Early studies have shown the vital role of NGF in the development and regeneration of sympathetic and sensory neurons [15]. Glial cell-derived neurotrophic factor (GDNF) family ligands (GFLs):- This family consists of homodimeric polypeptides: GDNF, neurturin (NRTN), artemin (ARTN), and persephin (PSPN), which also belong to the transforming growth factor β superfamily. GDNF is the first identified member in this family with an important role in the survival of midbrain dopaminergic neurons in a specific manner [16]. Neuropoietic cytokines (neurokines) family, the neuropoietic family includes ciliary neurotrophic factor (CNTF), leukemia inhibitory factor (LIF), interleukin-6 (IL-6), interleukin-11 (IL-11), cardiotrophin-1 (CT-1), cardiotrophin-like cytokine (CLC), neuropoietin, and oncostatin-M (OSM) [17].
Neurotrophic factor regulation of neuronal cell survival in the developing nervous system and promotion of survival after injury or protection of neurons in toxin-mediated disease animal models has been the underlying drive for the idea that neurotrophic proteins could be harnessed to treat neurodegenerative disease. For example, the traditional perspective of applying neurotrophins in the context of Alzheimer's disease is based on the premise that these polypeptides are capable of upregulating cholinergic function and rendering neurons less vulnerable to at least some processes causing neurodegeneration [18, 19]. Another example is GDNF, which acts on dopaminergic neurons. Administered intracerebrally, GNDF has neuroprotective and neurorestorative effects in toxin-induced rodent and nonhuman primate models of Parkinson's disease [20-22].
Neurotrophic factor treatment of CNS diseases presents an especially complex problem, given their poor pharmacokinetics and bioavailability, together with their inability to cross the blood-brain barrier. Neurotrophic proteins can be modified to increase blood-brain barrier transport [23], and intravenous administration of BDNF, conjugated to an anti-transferrin, can traverse the blood-brain barrier in rats and provide neuroprotection in focal transient brain ischemia but in large primates this effect is debatable [24, 25]. Using gene therapy as an enabling delivery technology, a number of review studies suggested the continued use of neurotrophic factors for treating a variety of neural-related illnesses, such as AD, PD, ALS, optic nerve degeneration, and hearing loss [26]. Research indicates that neurotrophic factors may have neuroprotective effects, but there are still a number of obstacles to overcome. When developing therapeutic techniques, it is important to take into account the numerous signaling networks, the blood-brain barrier, and the intricacies of the central nervous system.
Review Methods
This review focuses on clinical studies concerning the neuroprotective effect of neurotrophic factors in neurodegenerative diseases. We excluded animal studies and publications that discussed only the methodology of neurotrophic factors without presenting clinical data. The review follows the guidelines for Preferred Reporting Items for Systematic Reviews and Meta-Analyses (PRISMA) [27] for 2020 in Figure 2 and only uses data collected from published papers, eliminating the need for ethical approval.
Figure 2: PRISMA flow diagram illustrating the search strategy and study selection process for the systematic review. PRISMA: Preferred Reporting Items for Systematic Reviews and Meta-Analyses.
Systematic Literature Search and Study Selection
We conducted an intricate search for relevant publications by using PubMed, including Medline and Google Scholar. We searched for studies mentioned in review papers, editorials, and commentaries on PubMed. Nevertheless, we continued searching for additional studies that satisfied our inclusion criteria. We had a list of abstracts that we independently reviewed for inclusion using specific criteria. emphasizing their neuroprotective benefits in neurodegenerative disorders, availing neurotrophic factors, and having a well-described clinical cohort in the study were among the prerequisites. Research and review articles involving animals were not included. Controversies were settled through discussions following a dual review by six reviewers.
Inclusion and Exclusion Criteria
We established specific criteria for including and excluding participants to achieve our study goals. Our criteria can be summarized in (Table 1).
Inclusion criteria |
Exclusion criteria |
Human studies |
Animal studies |
From 2013 to 2023 |
Only methodological studies explaining programming details |
English Text |
Non-English texts |
Gender |
All |
Age: > 13 years of age |
Age:<=13 years |
Free papers |
Papers that needed to be purchased |
|
Studies involving clinical data other than neurodegenerative diseases |
Table 1: Showing the criteria adopted during the literature search process.
Search Strategy
A comprehensive review of the literature was carried out using the PICO (population, intervention/condition, control/comparison, and outcome) criteria. The relevant keywords for the search were neurotrophic factors, neuroprotective effects, and neurodegenerative illnesses. The databases searched were PUBMED (including Medline) and Google Scholar Libraries. Detailed search strategy was evolved using the medical subject heading (MeSH) approach for PubMed (including Medline) and Google Scholar, as described in (Table 2).
Database |
Search Strategy |
Search Results |
PubMed |
Neurodegenerative diseases |
1248 |
|
Neurotrophic Factors or neurodegenerative diseases |
94 |
|
Neurotrophic factors or neurodegenerative diseases or Neuroprotective effects |
77629 |
Google Scholar |
Neurotrophic factors or neurodegenerative diseases or Neuroprotective effects |
4146 |
Table 2: Showing the search strategy, search engines used, and number of results displayed.
Quality Appraisal
Several quality assessment tools were used to verify the dependability of the selected papers. For randomized clinical trial evaluation in systematic reviews and meta-analyses, we used the PRISMA checklist and the Cochrane bias tool. Using the Newcastle-Ottawa tool scale, non-randomized clinical studies were assessed. Using the Critical Appraisal Skills Program (CASP) checklist, qualitative research were evaluated (see Table 3). In order to preserve classification clarity, we assessed the articles' quality using the Scale for Assessment of Narrative Review Articles (SANRA).
Quality Appraisal Tools Used |
Type of Studies |
Cochrane Bias Tool Assessment |
Randomized Control Trials |
Newcastle-Ottawa Tool |
Non-RCT and Observational Studies |
PRISMA Checklist |
Systematic Reviews |
SANRA Checklist |
Any Other without Clear Method Section |
Table 3: Showing quality appraisal tools used.
PRISMA: Preferred reporting items for systematic reviews and meta-analyses; SANRA: Scale for the Assessment of Non-Systematic Review Articles.
Results
We extracted 80,226 articles after reviewing three specific databases: PubMed, Medline, and Google Scholar. After carefully going over each paper and applying particular criteria, 76,094 publications were excluded from consideration. 3,919 of the 4,132 papers that were still available were not used by us because of duplication or poor titles and abstracts. Our inclusion criteria were not met by the remaining 213 publications, so we carefully reviewed them and eliminated another 183. After that, we gave the final eight papers-all of which satisfied our requirements—a careful quality review. In our final systematic review, these eight articles are included. A full description of each is given in (Table 4).
Author/Year |
Country |
Study Design |
Database Used |
Conclusion |
Skaper SD/ 2018(14) |
Italy |
Systematic literature review |
Pubmed |
They answered the importance of neurotrophic factors |
RT/ 2017 (26) |
USA |
Systematic review |
Pubmed |
Breakthroughs with neurotrophic factors in neurodegenerative diseases |
Bali p/ 2017(31) |
India |
Systematic review |
PubMed |
They answered the key role of neurotrophic factors. |
Huang Y/ 2018 (36) |
China |
Systematic review |
PubMed |
BDNF concentration was positively associated with disease duration, severity of PD symptoms, and treatment with L-DOPA. |
Choi, S.H/ 2018(50) |
USA |
Randomized control trial |
Google Scholar |
Exercise-induced AHN (Adult Hippocampus Neurogenesis) improved cognition along with reduced Aβ load and increased levels of BDNF, IL-6, FNDC5, and synaptic markers. |
Karimi, N/ 2022(52) |
Iran, USA |
Systematic review and meta-analysis |
Google Scholar |
Circulating levels of BDNF are decreased in MS. |
Braschi, C/ 2021 (55) |
Italy |
Randomized control trial |
Google Scholar |
Reinforces the potential therapeutic ses of BDNF in neurological disorders and the potentiality of the non-invasive intranasal route as a brain delivery strategy for BDNF or other neurotrophic factors. |
Miranda, M/ 2019 (56) |
Argentina |
Systematic review |
PubMed and Google Scholar |
BDNF can be thought of as a marker that specifically relates to the occurrence and/or progression of the mnemonic symptoms that are common to many pathological conditions that share deficits in this cognitive domain. |
Table 4: Summary of the results of the selected papers.
Discussion
Novel therapeutic approaches are in trials to slow down neuronal deterioration and prolong neuronal life, ultimately aiding in the healing process. NTFs have a key physiological role in neuroprotective mechanisms and neurorestoration, suggesting they have a lot of potential for treating disorders linked to them [28].
For example, the decrease in NGF levels in Alzheimer’s patients [29] and the decrease in BDNF levels in a few regions of the brain, such as the substantia nigra, in Parkinson’s patients [30] ultimately lead to a deprivation in neuronal function preceded by a decrease in the number and size of neurons. Since CREB (cAMP response element-binding protein) is a DNA-binding protein and acts as a transcription factor for several genes, including c-fos, tyrosine hydroxylase, several neuronal peptides, and, importantly, neurotrophin BDNF, it is possible that an association may exists between the role of BDNF expression and its regulation by CREB in rescuing learning and memory deficits [31].
BDNF acts on cells by binding to either the tyrosine kinase receptor (Trk) B or the low-affinity p75 neurotrophin receptor (p75), a member of the tumor necrosis factor receptor superfamily [31]. BDNF can also bind to the Tropomyosin receptor kinase B (TrkB) and p75 receptors.Survival-promoting effects of BDNF are mediated by the TrkB receptor through activation of MAPK, Akt, and phospholipase C- pathways, while p75 receptors are mainly activated by the BDNF precursor molecule, proBDNF, triggering pro-apoptotic activity [40]. Interestingly, p75 seems to be upregulated in neurodegenerative disorders and aging [33]. In human PD patients, BDNF levels were found to be decreased in the SNPC [34] and in the serum [35]. However, a quite surprising increase in BDNF levels in the later stages of the disease was also reported [36]. BDNF promotes the survival of dopamine neurons in vitro and in animal models of PD [37-39].
The mature form of BDNF exerts its neuroprotective effects through TrkB, activating mitogen-activated protein kinase (MAPK), phosphatidylinositol-4,5-bisphosphate 3-kinase (PI3K)/Akt, and phospholipase C (PLC) pathways. Alternatively, pro-BDNF can exert pro-apoptotic effects through the p75 receptor. GFLs: GDNF, NRTN, ARTN, and PSPN, act mainly through receptor tyrosine kinase RET together, requiring additional coreceptors GDNF Family Receptor alpha 1 to 4 (GFRα1-4). GDNF, NRTN, ARTN, and PSPN bind to GFRα1 to 4, respectively. The binding of the GFL-GFR complex to RET activates MAPK, Src, and PI3K/Akt signaling pathways. Additionally, GFLs can also signal through GFR-NCAM and Syndecan 3 (except for PSPN in the latter case). CDNF is an unconventional neurotrophic factor without a known membrane receptor. Putatively, CDNF can act both on the plasma membrane and intracellularly on the ER membrane. It exerts prosurvival effects by attenuating ER stress and interacting with misfolded proteins [40].
In the case of potential therapy for neurodegenerative diseases, GDNF has a relatively high specificity for dopaminergic neurons and, thus, has significant potential for the treatment of PD, which is mainly characterized by progressive depletion of dopaminergic cell populations in the midbrain [41]. GDNF is the most studied NTF in PD. GDNF acts on neurons through its receptor, tyrosine kinase rearranged during transfection (RET), in the presence of a co-receptor, GDNF Family Receptor α1 (GFRα1). RET activation triggers complex intracellular signaling cascades, promoting the survival and regeneration of neurons [42]. In addition, GDNF has been demonstrated to possess trophic and protective properties on noradrenergic neurons located in the locus coeruleus, as well as on peripheral motor neurons. These findings raise optimism regarding the potential therapeutic applications of GDNF in treating Huntington's disease (HD) and amyotrophic lateral sclerosis (ALS) [43, 44, 45]. CNTF affects motor neuron survival in vitro, during development, after injury to motor neuron systems, and in genetic models of motor neuron degeneration [46]. This suggests that CNTF could be developed as a treatment for ALS [47] and SCI [48], where there is widespread degeneration of ventral motor neurons [49].
Research examining disease models of Alzheimer's disease (AD) has shown that boosting brain-derived neurotrophic factor (BDNF) by means of lentivirus expression of BDNF is essential for multiple functions in adulthood, such as cholinergic innervation, memory acquisition, and retention [50, 51]. Reduced amounts of BDNF have been found in the brains, blood, and cerebrospinal fluid (CSF) of patients with AD and moderate cognitive impairment [51]. In a similar vein, poor BDNF secretion in the serum is seen in people with multiple sclerosis (MS), which may also lead to decreased neuroprotection [52, 53]. As a result, it is expected that lower BDNF levels will impede MS patients' ability to enter remission and accelerate the disease's progressive stage [54]. Thus far, studies have looked into the possible advantages of BDNF in a range of inflammatory and neurodegenerative disorders, such as animal models of AD and spinal cord, MS, and Huntington's disease (HD) [55-57].
Limitations
This review of the literature has limitations. We looked for articles with an minimum age of 13 years old and constrained analysis to English publications released in the previous 10 years. Our analysis was constrained to English studies on neurotrophic factors and their neuroprotection in neurodegenerative illnesses, and we only used free literature. Precise inferences can be made by further research.
Conclusion
This systematic analysis draws the inference from the existing potential of neurotrophic factors as impressive neuroprotective treatments in neurodegenerative illnesses. Their wide-ranged roles in fostering neuronal survival, growth, and support are illustrated by a thorough analysis of the literature. Although administration, dosage, and long-term effectiveness, are still issues, the study clearly indicates that neurotrophic factors are the secret to pioneering therapeutic approaches. Although non-human primates benefit from neurotrophic factors, getting these factors into target neurons is still quite difficult since molecules are not very good at penetrating through peripheral channels. Administering medication intraventricularly or intrathecally only affects the cortex's outer layers and has negative side effects. Innovatory neurosurgical procedures have not yet been used to investigate the efficacy of this intriguing alternative approach to delivering BDNF in Parkinson's disease (PD) by gene transfer using viral vectors [58,59]. For these discoveries to be interpreted into physical therapies that can lessen the effects of neurodegenerative diseases and improve the survival of those who are impacted, more investigation and clinical trials are necessary.
Declarations
Ethical Approval and Consent to participate
Not Applicable
Consent for publication
Not Applicable
Availability of supporting data
Not Applicable
Competing Interest
Not Applicable
Source of Funding
None
Authors' contributions
Dr Rizwana Syed- Abstract, Review
Dr Niharika Reddy- Discussion
Dr Sarang Tusharbhai Mehta- Introduction and background
Dr Binay Panjiyar- Results
Dr Amir Rasheed- Conclusion
Acknowledgements
We would like to express our profound gratitude to everyone, who helped us finish this systematic review. Most importantly we would like to express our sincere gratitude to all of the authors in the studies that were part of this review. Their enthusiasm to share their knowledge and perspectives has been extremely helpful in uplifting our perception of how neurotrophic factors protect neurons in neurodegenerative diseases. We sincerely thank our team for their efforts, hard work, dedication, and commitment at every level of this review. Their knowledge, conscientiousness, and support have been crucial to ensuring the accuracy and breadth of our analysis.
References
- Liu H, Hu Y, Zhang Y, Zhang H, Gao S, et al. (2022) Mendelian randomization highlights significant difference and genetic heterogeneity in clinically diagnosed Alzheimer’s disease GWAS and self-report proxy phenotype GWAX. Alzheimer’s Res Ther. 14:17.
- Jain N, Chen-Plotkin AS. (2018) Genetic modifiers in neurodegeneration. Curr Genet Med Rep. 6(1):11-9.
- Jain V, Baitharu I, Barhwal K, Prasad D, Singh SB, et al. (2012) Enriched environment prevents hypobaric hypoxia induced neurodegeneration and is independent of antioxidant signaling. Cell Moll Neurobiol. 32(4):599-611.
- Przedborski S, Vila M, Jackson-Lewis V. (2003) Series Introduction: Neurodegeneration: What is it and where are we? J Clin Invest. 111(1):3-10.
- Martin JB. (1999) Molecular basis of the neurodegenerative disorders. N Engl J Med. 340(4):1970-80.
- Mattson MP. (2000) Apoptosis in neurodegenerative disorders. Nat Rev Mol Cell Biol. 1:120-30.
- Hague S, Klaffke S , Bandmann O. (2005) Neurodegenerative disordersParkinson’s disease and Huntington’s disease. J Neurol Neurosurg Psychiatry. 76 (8): 1058–63.
- Harding BN, Kariya S, Monani UR, Chung WK, Benton M, et al. (2015) Spectrum of neuropathophysiology in spinal muscular atrophy type I J Neuro Pathol Exp Neurol. 74(1):15-24.
- Klockgether T, Mariotti C, Paulson HL. (2019) Spinocerebellar ataxia. Nat Rev Dis Primers. 5:1-21.
- Merelli A, Czornyj L, Lazarowski Al. (2013) A neuroprotective agent in cerebral hypoxia, neurodegeneration, and epilepsy. Curr Pharm Des. 19(38):6791-801.
- Choonara YE, Pillay V, Du-Toit LC, Modi G, Naidoo D. (2009) Trends in the molecular pathogenesis and clinical therapeutics of common neurodegenerative disorders. Int J Mol Sci. 3;10 (6):2510-57.
- Rapp T, Chauvin P, Costa N, Molinier L. (2015) Health economic considerations in neurodegenerative disorders. Imaging Neuro degener Disord. 2:54.
- Levi-Montalcini R. (1987) The nerve growth factor 35 years later. Science6(5):1145-4.
- Skaper SD. (2018) Neurotrophic Factors: An Overview. Methods Mol Biol. 1727:1-17.
- Maness LM, Kastin AJ, Weber JT, zadina JE, Beckman BS, et al. (1994) The neurotrophins and their receptors: Structure, function, and neuropathology. Neurosci Biobeha Rev. 18 (1):143-59.
- Lin LH, Doherty DH, Lile JD, Beketsh S, Collins F, et al . (1993) GDNF: A Glial Cell Line-Derived Neurotrophic Factor for Midbrain Dopaminergic Neurons. Science;260(5111) :1130-2.
- Carnow TB, Manthorpe M, Davis GE, Varon S, et al. (1985) Localized survival of ciliary ganglionic neurons identifies neuronotrophic factor bands on nitrocellulose blots. J. Neurosis. 5(8):1965-71.
- Hefti F. (1986) Nerve growth factor promotes survival of septal cholinergic neurons after fimbrial transactions. J Neurosci. 6(8):2155-62.
- Williams LR, Varon S, Peterson GM, Wictorin K, Fischer W. (1986) Continuous infusion of nerve growth factor prevents basal forebrain neuronal death after f imbria fornix transaction. Proc Natl Acad Sci U S A 83(23):9231-5.
- Kordower JH, Emborg ME, Bloch J, Ma SY, Chu Y, et al. (2000) Neurodegeneration prevented by lentiviral vector delivery of GDNF in primate models of Parkinson’s disease. Science 290(5492):767-73.
- Yasuda T, Mochizuki H (2010) Use of growth factors for the treatment of Parkinson’s disease. Expert Rev Neurother 10(6):915-24.
- Domanskyi A, Saarma M, Airavaara M (2015) Prospects of neurotrophic factors for Parkinson’s disease: comparison of protein and gene therapy. Hum Gene Ther 26(8):550-9.
- Podulso JF, Curran GL, Gill JS (1998) Putrescine-modified nerve growth factor: bioactivity, plasma pharmacokinetics, blood- brain/nerve barrier permeability, and nervous system biodistribution. J Neurochem 71(4):1651-60 .
- Wu D, Pardridge WM (1999) Neuroprotection with noninvasive neurotrophin delivery to the brain. Proc Natl Acad Sci U S A 96(1):254-9.
- Zhang Y, Pardridge WM. (2001) Neuroprotection in transient focal brain ischemia after delayed intravenous administration of brain-derived neurotrophic factor conjugated to a blood-brain barrier drug targeting system. Stroke. 32(6):1378-84.
- Alves, S., Fol, R., Cartier, N. (2016). Gene therapy strategies for Alzheimer's disease: an overview. Hum Gene Ther. 27(2):100-7.
- Bartus RT, Johnson EM Jr. (2017) Clinical tests of neurotrophic factors for human neurodegenerative diseases, part 1: Where have we been and what have we learned? Neurobiol Dis. Jan;97(Pt B):156-168.
- Liberati A, Altman DG, Tetzlaff J, Mulrow C, Gotzsche P,cet al.: The PRISMA statement for reporting systematic reviews and meta-analyses of studies that evaluate health care interventions: explanation and elaboration. PLoS Med,21 6(7) :e1000100.
- Ruozi B, Belletti D, Bondioli L, Vita De A, Froni F, et al. (2012) Neurotrophic Factors and Neurodegenerative Diseases: A Delivery Issue. Int Rev Neurobiol. 102: 207-47.
- Salehi A, Delcroix J, Swaab DF. (2004) Alzheimer ’s disease and NGF signaling. J. Neural Transm. 111(3):323-45.
- Costa A, Peppe A, Carlesimo GA, Zabberoni S, Scalici F, et al. (2015) Brain-derived neurotrophic factor serum levels correlate with cognitive performance in Parkinson ’ s disease patients with mild cognitive impairment. Front Behav Neurosci . 15:9:253.
- Bali P, Lahiri DK, Banik A, Nehru B, Anand A. (2017) Potential for Stem Cells Therapy in Alzheimer's Disease: Do Neurotrophic Factors Play Critical Role? Curr Alzheimer Res. 14(2):208-20.
- Mitre M, Mariga A, Chao MV. (2017) Neurotrophin signalling: novel insights into mechanisms and pathophysiology. Clin Sci.1:131(1):13-23.
- 34. 34. 33. Josephy-Hernandez S, Jmaeff S, Pirvulescu I, Aboulkassim T, Saragovi HU. (2017) Neurotrophin receptor agonists and antagonists as therapeutic agents: an evolving paradigm. Neurobiol Dis. 97(ptB):139-155.
- Parain K, Murer MG, Yan Q, Faucheux B, Agid Y, et al. Reduced expression of brain-derived neurotrophic factor protein in Parkinson’s disease substantia nigra. NeuroReport. 1999. https://doi.org/10.1097/00001756-199902250-00021.
- Ziebell M, Khalid U, Klein AB, Aznar S, Thomsen G, et al. (2012) Striatal dopamine transporter binding correlates with serum BDNF levels in patients with striatal dopaminergic neurodegeneration. Neurobiol Aging. 33(12):428.e1-5.
- Huang Y, Yun W, Zhang M, Luo W, Zhou X. Serum concentration and clinical significance of brain-derived neurotrophic factor in patients with Parkinson’s disease or essential tremor. J Int Med Res. 46(4):1477-85.
- Hyman C, Hofer M, Barde YA, Juhasz M, Yancopoulos GD, et al. (1991) BDNF is a neurotrophic factor for dopaminergic neurons of the substantia nigra. Nature. 350(6315):230-2.
- Tsukahara T, Takeda M, Shimohama S, Ohara O, Hashimoto N. (1995) Effects of brain-derived neurotrophic factor on 1-methyl4-phenyl-1,2,3,6-tetrahydropyridine-induced parkinsonism in monkeys. Neurosurgery. 37(4):733-41.
- Levivier M, Przedborski S, Bencsics C, Kang UJ. (1995) Intrastriatal implantation of fibroblasts genetically engineered to produce brain-derived neurotrophic factor prevents degeneration of dopaminergic neurons in a rat model of Parkinson’s disease. J Neurosci. 15(12):7810-20.
- Chmielarz P, Saarma M. (2020) Neurotrophic factors for disease-modifying treatments of Parkinson's disease: gaps between basic science and clinical studies. Pharmacol. Rep 72(5):1195-217
- Lapchak PA, Jiao S, Collins F, Miller PJ. (1997) Glial cell line-derived neurotrophic factor: Distribution and pharmacology in the rat following a bolus intraventricular injection. Brain Res. 747(1):92-102.
- Airaksinen MS, Saarma M. (2002) The GDNF family: signalling, biological functions and therapeutic value. Nat Rev Neurosci. 3(5):383-94.
- Thomsen GM, Avalos P, Ma AA, Alkaslasi M, Cho N, et al. (2018) et al. (2018) Transplantation of Neural Progenitor Cells Expressing Glial Cell Line-Derived Neurotrophic Factor into the Motor Cortex as a Strategy to Treat Amyotrophic Lateral Sclerosis. Stem Cells. 36(7):1122-31.
- Suzuki M, McHugh J, Tork C, Shelley B, Klein SM, et al. (2007) GDNF secreting human neural progenitor cells protect dying motor neurons, but not their projection muscule, in a rat model of familial ALS. PLoS ONE. e689.
- Suzuki M, McHugh J, Tork C, Shelley B, Hayes A, et al. (2008) Direct muscle delivery of GDNF with human mesenchymal stem cells improves motor neuron survival and function in a rat model of familial ALS. Mol Ther. 16(12):2002-10.
- Cedarbaum JM, Chapman C, Charatan M, Stambler N, Andrews L, et al. (1995) A phase I study of recombinant human ciliary neurotrophic factor (rHCNTF) in patients with amyotrophic lateral sclerosis. Clin. Neuropharmacol. 18:515-32.
- Bongioanni P, Reali C, Sogos V. (2004) Ciliary neurotrophic factor (CNTF) for amyotrophic lateral sclerosis or motor neuron disease. Cochrane Database Syst Rev. 2004(3):CD004302.
- Talbott JF, Cao Q, Bertram J, Nkansah M, Benton RL, et al. (2007) CNTF promotes the survival and differentiation of adult spinal cord-derived oligodendrocyte precursor cells in vitro but fails to promote remyelination in vivo. Exp. Neurol. 204(1):485-9.
- Abbaszadeh HA, Tiraihi T, Noori-Zadeh A, Delshad AR, Sadeghizade M. et al. (2015) Human ciliary neurotrophic factor-overexpressing stable bone marrow stromal cells in the treatment of a rat model of traumatic spinal cord injury. Cytotherapy. 17(7):912-21.
- Choi SH, Bylykbashi E, Chatila ZK, Lee SW, Pulli B, et al. (2018) Combined adult neurogenesis and BDNF mimic exercise effects on cognition in an Alzheimer’s mouse model. Science 2018, 361(6406):eaan8821.
- Blurton-Jones M, Kitazawa M, Martinez-Coria H, Castello NA, Müller FJ, et al. (2009) Neural stem cells improve cognition via BDNF in a transgenic model of Alzheimer disease. Proc Natl Acad Sci USA. 106(32):13594-9.
- Karimi N, Ashourizadeh H, Akbarzadeh B, Haghshomar M, Jouzdani T, et al. (2022) Blood levels of brain-derived neurotrophic factor ( BDNF ) in people with multiple sclerosis ( MS ): A systematic review and meta-analysis. Mult. Scler Relat Disord. 65(103984.
- Azoulay D, Urshansky N, Karni A. (2008) Low and dysregulated BDNF secretion from immune cells of MS patients is related to reduced neuroprotection. J Neuroimmunol. 195(1-2):186-193.
- Knaepen K, Goekint M, Heyman EM, Meeusen R. (2010) Neuroplasticity-Exercise-Induced Response of Peripheral Brain-Derived Neurotrophic Factor A Systematic Review of Experimental Studies in Human Subjects. Sport Med. 40(9):765-801.
- Braschi C, Capsoni S, Narducci R, Poli A. (2021) Intranasal delivery of BDNF rescues memory deficits in AD11 mice and reduces brain microgliosis. Aging Clin Exp Res. 33(5):1223-38.
- Gransee HM, Zhan WZ, Sieck GC, Mantilla CB. (2015) Localized delivery of brain-derived neurotrophic factor-expressing mesenchymal stem cells enhances functional recovery following cervical spinal cord injury. J. Neurotrauma 2015, 32(3):185-93.
- Miranda M, Morici JF, Zanoni MB, Bekinschtein, P. (2019) Brain-Derived Neurotrophic Factor: A Key Molecule for Memory in the Healthy and the Pathological Brain. Front Cell Neuro sci. 2019, 13:363.
- Jimcy Platholi, Francis S, Lee. (2018) Chapter 5 - Neurotrophic Factors, Handbook of Developmental Neurotoxicology (Second Edition), Academic Press. Pages 55-64.
- Vivek Sudhakar R. (2019) Mark Richardson, Gene Therapy for Neurodegenerative Diseases, Neurotherapeutics. 16(1):166-75.