Micro fractured Adipose Tissue Graft (Lipogems), Regenerative Surgery and Potential Outcomes for Infectious and Cancer Diseases
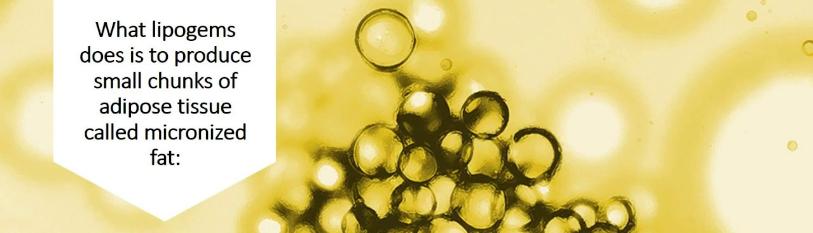
Carlo Tremolada1, Pierre Rocheteau2, Carmelo Bisognano2 *, Offer Zeira3, and Giulio Alessandri4
1Image Regenerative Clinic, Milano, Italy
2Exogems SA, c/o Biopole, Epallinges, Switzerland
3Department of Neurology, Neurosurgery and Imaging diagnostics, Stem Cells and Regenerative Medicine
San Michele Veterinary Hospital, Tavazzano con Villavesco, Italy
4StaMeTec coordinated Research Centre, Department of Biomedical, Surgical and Dental Sciences, University of Milan, Milan
*Corresponding author: Carmelo Bisognano, Exogems SA, c/o Biopole, Epallinges, Switzerland
Citation: Tremolada C, Rocheteau P, Bisognano C, Zeira O, Alessandri G. (2023) Micro fractured Adipose Tissue Graft (Lipogems), Regenerative Surgery and Potential Outcomes for Infectious and Cancer Diseases. J Stem Cell Res. 4(2):1-15.
Received: September 6, 2023 | Published: September 27, 2023.
Copyright© 2023 by Tremolada C. All rights reserved. This is an open access article distributed under the terms of the Creative Commons Attribution License, which permits unrestricted use, distribution, and reproduction in any medium, provided the original author and source are credited.
DOI: https://doi.org/10.52793/JSCR.2023.4(2)-53
Abstract
In the past few years, interest in adipose tissue as an ideal source of mesenchymal stem cells (MSCs) has increased since they are able to secrete many bioactive molecules and exosomes. MSCs are being used increasingly for many clinical applications, such as orthopedic, plastic, and reconstructive surgery. An innovative technique (Lipogems®) has been developed to obtain micro fragmented adipose tissue (MFAT) with an intact stromal vascular niche and MSCs with a high regenerative capacity. The Lipogems technology, is an easy-to-use system designed to harvest, process, and inject refined fat tissue and is characterized by optimal handling ability and a great regenerative potential based on adipose-derived MSCs. As a further step, bioactive molecules and exosomes produced either by the MSCs extracted and from Lipogems/MFAT itself will be studied by Exogems Inc., a new venture that will leverage the potential of this secretome to treat infections and cancer.
Keywords
Adipose tissue; Exosomes; Mesenchymal cells; Cancer; Infections
Introduction
The adipose tissue (AT), encompassing subcutaneous and visceral fat, constitutes the largest organ within our body and serves a range of functions, both in terms of structure and endocrine activity [1]. This tissue comprises various cellular elements (depicted in Figure 1 and possesses an intricate network of small capillaries that actively engage in the body's self-repair mechanisms. Notably abundant in pericytes (illustrated in Figure 2), which serve as precursors to mesenchymal stem cells (MSCs), crucial components in the healing process [2]. Throughout history, fat has been harnessed for medicinal purposes, with applications dating back to military physicians in the Napoleonic era. However, it was not until the 1980s that the liposuction technique emerged, revolutionizing access to sizable fat deposits using progressively less invasive methods. This paved the way for the popularity of lipofilling, an approach involving the autologous transplantation of adipose tissue. Initially used to restore lost volume, such as in breast reconstruction or facial deformities, its application expanded to regenerative purposes, particularly in addressing scars and radio dermatitis [3]. Despite delivering promising clinical outcomes, traditional lipofilling techniques encountered significant challenges, notably variations in results (volume absorption, residual irregularities, and fibrosis), as well as unpredictability in regenerative outcomes [1].
Figure 1: Adipose tissue is composed of many cellular components which can be separated by enzymatic treatment. These are found in the Stromal Vascular Fraction (SVF) [2].
Figure 2: (a) Pericytes (red cells) sit around the microvessels of all tissues and are the precursor of MSCs. Electronic microscopy showing pericytes in a 5-micron capillary. (b) Lipogems cluster (adipocytes are in orange and Pericytes are in green) showing the pericytes and the intact microvessels on the surface of the cluster [2].
Comparative analysis of adipose tissue and bone marrow aspirate as sources of biologically and clinically active Mscs for regenerative applications
Both AT and bone marrow (BM) have emerged as primary contenders for regenerative therapies in the musculoskeletal system, owing to their minimally invasive harvesting methods and the presence of MSCs in their aspirates. The scrutiny of MSCs originating from BM began with Arnold Caplan's pioneering investigations, building upon the established knowledge of BM as the precursor site for hematopoietic stem cells. Although BM derived MSCs took the lead in terms of study, isolation, and characterization, AT has shown to possess comparable regenerative attributes, boasting distinct advantages like abundance, even simpler harvesting procedures, and a substantially larger yield of MSCs [4-5]. The functional and clinical impact of MSCs predominantly hinges on their secretome, encompassing exosomes, proteins, and RNAs. This secretome is so influential that Caplan himself coined the term "Medicinal Signaling Cell" to underscore their pivotal biological role in maintaining tissues and responding to injuries, particularly by modulating inflammation and macrophage activity. Following a traumatic event to a tissue's capillary wall, pericytes detach and transform into MSCs within minutes to hours, releasing a plethora of cytokines that impede inflammation, especially induced by macrophages [6]. In the subsequent weeks, more specialized cytokines are secreted, fostering antibiotic, antifibrotic, angiogenetic, and analgesic effects [6]. This field of medical research is experiencing a surge, evident from over 8500 literature entries in the past year alone.
The specific density of MSCs required to induce certain biological responses remains unclear. Nevertheless, the efficacious clinical outcomes observed in symptomatic osteoarthritis treatment using concentrated bone marrow aspirate (BMAC), containing approximately 20,000 MSCs per cubic centimeter, closely rival the results from lipogems at one year. Notably, lipogems boasts a significantly higher average content of about 1,000,000 MSCs per cubic centimeter. While counting MSCs directly in bone marrow is comparatively straightforward, doing so in AT poses challenges due to the majority of MSCs being sensnared within micro vessels present in tissue clusters. Counting MSCs in AT necessitates enzymatic or mechanical separation processes, which entail either complete (enzymatic) or partial (mechanical) disruption of adipose tissue and its inherent structure. This yields a cellular amalgam known as the stromal vascular fraction (SVF), extensively employed in clinical and laboratory research, yet subject to notable regulatory issues in standard clinical practice. Interestingly, SVF from 1 cubic centimeter of adipose tissue contains around 500 times more MSCs compared to 1 cubic centimeter of unconcentrated bone marrow aspirate [7]. None the less, as observed in clinical trials, there exists a modest correlation between the quantity of MSCs and the ensuing biological and clinical effects [7].
The preceding discussion underscores a critical point: Lipogems is unequivocally not a device designed for the mechanical isolation of MSCs, a common misconception held by many [8-10]. Contrary to this misunderstanding, MFAT, the outcome of Lipogems, stands in stark contrast to the stromal vascular fraction (SVF) generated through enzymatic or mechanical breakdown of the lipoaspirate, which necessitates complete dismantling of the original tissue structure to facilitate potential MSC isolation and cultivation. The Lipogems extract represents AT that has been substantially reduced in size and thoroughly cleansed from a lipoaspirate, maintaining clusters of approximately 3mm. It is crucial to grasp that this extract remains authentic AT, still containing fully intact clusters measuring around 0.3/0.5 mm. Of notable significance is the fact that the surface of these clusters is rich in microvessels, presenting an exposure that is considerably amplified (roughly 6000 times greater) when compared to the initial lipoaspirate [11]. Recognizing the distinctions between various regenerative preparations holds immense importance in comprehending their divergent biological and clinical impacts. Notably, it remains far from certain, as previously mentioned, that a higher quantity of MSCs invariably leads to more pronounced biological effects. This applies to both the secrete and exosomes obtained in vitro, as well as the evaluation of final biological effects in animal studies conducted in vivo [12-13].
The Lipogems Device and the Resemblance of MFAT (Lipogems) to Lipoaspirate, with Enhanced Biological Attributes, Rendering it a Natural Implantable Bioreactor
Originally conceived and patented with the aim of optimizing clinical outcomes in lipofilling, the Lipogems system (depicted in Figure 3) operates by aspirating adipose tissue using small, disposable cannulas (14G) featuring multiple oval apertures sized at 2x1 mm. This choice of aperture dimensions, while smaller, avoids procedural delays and excessive damage to the aspirated fat. Subsequently, the aspirated adipose tissue, characterized by clusters around 3 mm in size, undergoes processing within a completely sealed device. Within this device, the fat is meticulously washed to remove blood, oily residues, and inflammatory components. Concurrently, the adipose clusters are gradually diminished in size to approximately 0.3 mm using precise mechanical forces applied through sharp filters. The correct execution of this process is pivotal, as emphasized in the Lipogems tutorial an especially critical factor is the exclusion of air during the procedure. This ensures that volumetric reduction occurs while harnessing the protective influence of physiological pressure, preventing the adipocytes from rupturing and preserving the structural integrity of the adipose tissue. The marbles contained within the device serve not to fragment the adipose tissue, but to facilitate effective cleansing against the gravitational forces of the oily and blood emulsion. They also contribute to dispersing the tissue, enabling it to pass smoothly through the subsequent cutting filter. During this process, shaking should be carried out for a duration lasting no less than 20 seconds and no more than 90 seconds, all the while assuring the absence of air within the device. This meticulous procedure contributes to the successful transformation of the AT into MFAT, commonly referred to as Lipogems. It is important to recognize that this MFAT, despite its resemblance to lipoaspirate, showcases enhanced biological properties, to the extent that it can be deemed a natural implantable bioreactor.
Figure 3: The Lipogems system [2].
The Lipogems product (depicted in Figure 4) embodies a tissue fragment that has been meticulously cleansed of oily residues and deceased cells, preserving its structural integrity at a diameter of around 300 microns (0.3 mm). This product essentially serves as a natural implantable bioreactor, encompassing the essential components for an optimal natural regenerative response: the scaffold (notably integrating seamlessly even at the microvascular level of adipose tissue), the cells (particularly pericytes/MSCs), and the growth factors (cytokines secreted in response to mechanical trauma and subsequently tailored to the implantation environment of Lipogems clusters). When this tissue is locally injected, it functions as a living graft that seamlessly integrates with the recipient tissue. Its modest size and structural integrity render it an ideal graft, facilitating both swift passive and active revascularization processes. This, in turn, enable it to effectively serve over an extended period, considerably enhancing the inherent potential for local healing. Pertinently, it is intriguing to observe how individual recovery following trauma or illness differs significantly due to the vascular micro-density of tissues [14-15]. This micro-density varies inversely with age and overall health conditions, evidenced by decreased vascular micro-density in conditions such as diabetes and in elderly tissue, as opposed to pediatric tissue.
Figure 4: The MFAT derived from Lipogems [2].
The process of downsizing the lipoaspirate from a diameter of 3mm to 0.3mm engenders a substantial escalation in the quantity of grafted fragments: each initial fragment gives rise to approximately 1000 fully intact pieces, each adorned with a surface layer of capillaries. Notably, this augmented surface area experiences an even more substantial enlargement, reaching up to around 6000 times its initial size. Consequently, an extensive network of capillaries, accompanied by a substantial presence of pericytes poised to naturally func- 174 tion, becomes exposed. Furthermore, investigations have demonstrated that Micro-Fragmented Adipose Tissue (MFAT) actively generates microcapillaries in vitro, akin to the process observed in an aorta ring [16]. Intriguingly, MFAT also stimulates neovasculogenesis within the recipient tissue (as depicted in Figure 5) [17].
Figure 5: Vessel-like structures sprouting from lipogems cluster in culture [2].
The information presented above underscores a crucial mechanism through which MFAT augments the healing potential of tissues, primarily by establishing a lasting enhancement in vascular density. This phenomenon leads to tangible tissue rejuvenation, a fact substantiated both clinically and through histological examinations of tissues treated in gynecological and aesthetic contexts, even several years post-application [17-20]. The transplantation of MFAT using the Lipogems technique, has been extensively and effectively employed in thousands of patients across diverse medical fields, spanning human and veterinary medicine. This method has been applied in various clinical scenarios for over 8 years, demonstrating its safety and efficacy [20-39].
The Regulatory Framework
The Lipogems device obtained FDA clearance in 2014 as a class II medical device designated for the processing of autologous AT. According to the FDA,s criteria, lipogems falls under the definition of tissue that is: (1) autologous; subject to minimal manipulation; (3) intended for homologous use (structural applications); (4) devoid of enzymes; (5) not reliant on cellular metabolic activity for its primary function; (6) utilized within the same surgical procedure; and (7) combined solely with saline, without any ad additional substances. In 2016, the FDA issued a secondary authorization for the processing 199 of adipose tissue for various surgical purposes, encompassing applications like arthroscopy. This signifies that MFAT can be safely utilized by surgeons as an autologous transplant in musculoskeletal scenarios and within joint areas. However, it's important to note that unlike drugs, medical devices do not mandate specific therapeutic indications within the regulatory framework. For instance, there is no explicit therapeutic indication stipulated for MFAT derived from the Lipogems device, such as for the treatment of osteoarthritis. Furthermore, both the FDA and the European Medicines Agency (EMA) categorize MFAT as an autologous transplant rather than a biological drug. Consequently, the therapeutic guidelines are governed by the principles of sound medical-surgical practice. These guidelines stem from indications derived from the results of clinical trials where the Lipogems/MFAT product has been established as safe and remarkably effective.
Clinical Applications of Lipogems
In the realm of general surgery, Lipogems has demonstrated its efficacy as a promising approach for addressing fecal incontinence and anal fistulas. Remarkably, even in irradiated environments, it has exhibited impressive morphological rejuvenation and the restoration of muscle and neurological function over the long term [33]. In orthopedic surgery, particularly within the musculoskeletal domain, a multitude of patients grappling with degenerative and inflammatory joint conditions have derived benefits from intra and periarticular Lipogems treatment. An expansive body of more than 140 continuously updated clinical studies attests to this fact. Post the injection of varying Lipogems quantities (ranging from 0.2 to 12 ml, contingent on the joint), patients have commonly reported both immediate alleviation of symptoms and enduring relief from pain. Notably, a significant portion of individuals previously earmarked for surgical intervention due to the severity of degeneration have found surgery to be unnecessary, thanks to the complete or substantial resolution of their symptoms. Comparative analyses of preoperative and postoperative radiographic and MRI findings often reveal signs of possible articular cartilage regeneration and an expansion of the joint space within 6 to 18 months post-treatment. However, it's essential to acknowledge that while clinical enhancement is frequently more pronounced than radiographic progress, sometimes there's minimal correlation between radiological and clinical observations. Moreover, Lipogems has proven to be advantageous in orthopedics to facilitate the healing of ligaments or tendons that are injured or degenerated, meniscal tears, around surgical incisions, within or around osteotomy sites, and in cases of delayed bone union. These favorable outcomes extend into the realm of Veterinary Medicine, where Lipogems has emerged as a noteworthy regenerative therapy, not only for dogs and horses but also for exotic animals [21].
In the realm of reconstructive plastic surgery, Lipogems plays a pivotal role in fostering healing of chronic leg and foot ulcers, particularly in diabetic patients. Encouraging results have been documented, demonstrating its potential [36]. Cosmetic surgery is an expanding domain of application for Lipogems, where it is used independently or in conjunction with traditional surgical methods like facelifts, eyelid surgeries, and breast augmentations. The prevailing sentiment is that Lipogems not only enhances but also expedites the healing process. Notably exceptional outcomes have been achieved in the periorbital region and within orthognathic procedures. Patients post-surgery frequently report minimal discomfort, swelling, or bruising, and are typically highly content with continuous improvements in skin texture and elasticity, particularly notable between 9 and 18 months post-operation. This mirrors the pattern observed across other surgical disciplines, where a robust early anti-inflammatory effect (manifesting in hours to days) is followed by a prolonged and regenerative effect (spanning months to years) [21].
Future Directions
Lipogems has the potential to serve as a reservoir of cultivated Mesenchymal Stem Cells (MSCs), exhibiting superior quality compared to those obtained through mechanical dissociation. This superiority extends to both differentiation potential and the composition of secretome. Notably, in the realm of in vivo applications, Lipogems outperforms both Stromal Vascular Fraction (SVF) and culture-expanded MSCs in the context of cartilage regeneration [41-42] (as illustrated in Figure 6). Furthermore, Lipogems has gained recent acceptance from the regional tissue bank in the Emilia Romagna region of Italy. It has been recognized as an autologous source of adipose tissue suitable for long-term storage, intended for deployment in regenerative procedures 261 or to be blended with other cryopreserved tissues.
Figure 6: An experimental model involving rabbit osteoarthritis vividly demonstrates the absence of any direct link between the quantity of MSCs and regenerative capability. Intriguingly, in this context, the quantitative disparities in cartilage regeneration among Micro-Fragmented Adipose Tissue (MFAT), Stromal Vascular Fraction (SVF), and cultured MSCs are practically negligible. In fact, upon histological scrutiny, it becomes apparent that the neo-cartilage originating from MFAT follows a more structurally normal trajectory, adhering to the natural maturation phases [42].
In addition to its application in reconstructive surgery, the Lipogems device is poised to play a role in other domains. Its future utility encompasses the treatment of sepsis and surgery site infections, as well as its potential as a drug delivery mechanism. Lipogems exhibits characteristics that position it as an ideal scaffold for localized drug delivery, which holds particular promise for addressing localized cancer treatment [43].
Exogems, The Next Quantum Leap of Lipogems
Exogems Inc. is a new venture leveraging Lipogems® technology to expand its potential for new indications (initially infections and cancer). Mesenchymal stem cells (MSCs) isolated with Lipogems (Lipogems-MFAT) have demonstrated to hold great potential for the treatment of several diseases. MSCs therapeutic potential is achieved by paracrine factors and the transfer of exosomes [44] (both referred as secretome) as a mean of communication and regulation among different cells (Figure 7).
Figure 7: Secretion of Exosomes by MSC [45].
Those exosomes are small particles (nanoparticles) with a diameter between 50nm and 150nm serving as carriers for key molecules (RNA, lipids, active molecules) to achieve a good communication between the cells [46] (Figure 8). Exosomes are crucial messengers that present in biological fluids and are involved in multiple physiological and pathological processes [47]. Exosomes were shown to have very promising effect on immunological diseases and regeneration of tissues and Exogems will use Lipogems extracted exosomes to treat sepsis and surgery-site infections [48], and brain cancer as a further approach. (no data for this application).
Figure 8: Detailed view of Exosomes by MSC [49].
Surgical Site Infections
Despite improvements in prevention, surgical site infections (SSIs) remain a significant clinical problem as they are associated with substantial mortality and morbidity and impose severe demands on healthcare resources. SSIs are an increasingly important postoperative problem known to complicate 2% to 4% of all surgeries in Europe which translates to 543 149 cases per year [50] and in the United States, with an estimated 570 000 postoperative cases [51]. Overall, the cost of SSI is estimated up to $10 billion in EU in direct and indirect medical costs each year. It has been previously shown that MSCs exhibit antimicrobial properties. These effects have been demonstrated to be both direct (by secreting anti-microbial factors such as LL37, cathelicidins, lipocalin-2 and beta-defensins) and indirect (by stimulating the host inner immune system) [52]. The potential of MSC therapy for infection management is also supported by many studies which found that MSC enhanced survival in a mouse model of sepsis [14]. In addition, MSC have pro-regenerative, anti-inflammatory and immunomodulatory capacities that would be beneficial for the wound healing, including stimulation of angiogenesis, activation of resident stem cell populations, reduction in inflammation, recruitment of immune cells, and suppression of scarring.
Sepsis
Sepsis is a life-threatening disease with high mortality, around 30% [49], and morbidity in all the critical care units around the world [50]. In the most recent international consensus, sepsis is defined as “life-threatening organ dysfunction caused by a dysregulated host response to infection” [51]. After decades of research, and numerous pre-clinical and clinical trials, sepsis remain without a specific and effective pharmacotherapy and essentially the management remains supportive [52]. Severe sepsis is a complex syndrome produced by the response to a systemic infection. The infection produces a general inflammatory response, such as tachycardia, elevated white cell count and systemic release of pro-inflammatory cytokines, and this can lead to an acute organ dysfunction [53]. Sepsis is causing 11 million deaths per year worldwide according to the World Health Organization [54]. Recent studies have shown that MSCs isolated from Lipogems are very potent against sepsis [14].
Figure 9: Description of Lipogems and preclinical results [14].
The Lipogems technology was used to in very severe model of sepsis12 and an improvement in inflammatory score and in survival was observed [14] (Figure 9). Exogem’s goal will be to capitalize on the very promising results obtained on sepsis to create a new secretome and an off-the-shelf biologics to fight acute and long-term effects of septic shock.
Promises of Lipogems/MFAT as Drug Delivery System (DDS) for Human Cancers
Cancer is one of the major causes of death worldwide. Usual treatments for multiple human cancers are nowadays represented by chemotherapy. However, it is of common knowledge that many of the drugs used for the treatment of tumors usually produce harmful side effects to patients, and this aspect is due to their extreme systemic toxicity. In this context, MFAT obtained via Lipogems may play an important role as DDS in the treatment of solid tumors, particularly those with little or no therapeutic possibility such as central nervous system (CNS) tumors, as new approach to favor anticancer drug local ization. Lipogems-MFAT drug-carrier capability is observed both in viable (fresh autologous MFAT) or non-viable tissue. When the extracellular component of Lipogems-MFAT is taken out by freezing/thawing, the processing results is a devitalized micro fragmented adipose tissue (DMFAT) with no viable cells, removed adipose niche component and preserved connective architecture / adipocyte structure. Although viable cells are absent, DMFAT contains exosomes and different cellular debris, including proteins and DNA [59]. Therefore, both Lipogems-DFAT/MFAT can act as a natural scaffold to perform loco-regional treatment of solid tumors as showed in two preclinical studies.
In an orthotopic mice model of Neuroblastoma (NB) transplant, paclitaxel (PTX) 382 loaded into MFAT is preventing, or delaying, NB relapse when placed in the sur- 383 gical area of tumor resection, without any collateral toxicity [59]. Paclitaxel (PTX) loaded into MFAT showed to be effective in inhibiting glioblastoma (GBM) growth in vitro [59] and in-vivo for mesothelioma [60-61]. MFAT (or DFAT) represents a very innovative natural biomaterial able to carry and release anti-cancer molecules at the tumor site with minimal systemic toxicity, helping to fight human cancer. Moreover, the autologous treatment is a major key factor easing regulation of a therapy arising from Lipogems MFAT while treating tumors in different surgical areas.
Conclusions
AT is the ideal source but above all for the practical clinical use of the Mesenchymal Stem Cells contained therein since (i) it is easily accessible and collected through a minimally invasive surgical procedure, (ii) it can be found in quantity sufficient in most people and (iii) ensures an adequate amount of progenitor cells with good viability and minimally age-related differentiating potential. Lipogems technology naturally optimizes the healing and regenerative properties of adipose tissue by reducing the size of the adipose tissue clusters (MFAT), maintaining their structural and functional integrity without using enzymes, additives or centrifugations, but relying on the use of mild mechanical forces and exploiting the protection offered by saline which fills a completely closed system in the absence of air. As a further step, Exogems is a new venture that will explore the potential of MFAT (or DFAT) in different areas of interest such as infection and oncology. Future developments may provide off-the shelve treatments for those life-threatening diseases.
Conflicts of Interest
Carlo Tremolada is a founder of Lipogems International SpA. Carmelo Bisognano is CEO of Exogems SA. Pierre Rocheteau and Carlo Tremolada are shareholders of Exogems SA. Offer Zeira and Giulio Alessandri declare that they have no conflict of interest.
References
-
Tremolada C, Palmieri G, Ricordi C. (2010) Adipocyte transplantation and stem cells: plastic surgery meets regenerative medicine. Cell Transplant. 19(10):1217-23.
-
Tremolada C. (2022) Microfractured Adipose Tissue Graft (lipogems) and Regenerative Surgery. J Orthop Res Ther. 7:1210.
-
Simonacci F, Bertozzi N, Grieco MP, Raposio E. (2019) From liposuction to adipose-derived stem cells: indications and technique. Acta Biomed. 90(2):197-208
-
Mattar P, Bieback K. (2015) Comparing the immunomodulatory properties of bone marrow, adipose tissue, and birth-associated tissue mesenchymal stromal cells. Front Immunol. 6:560
-
Caplan AI, Correa D. (2011) PDGF in bone formation and regeneration: new insights into a novel mechanism involving MSCs. J Orthop Res. 29(12):1795-1803.
-
Caplan AI, Correa D. (2011) The MSC: an injury drugstore. Cell Stem Cell. 9(1):11-15.
-
Mautner K, Bowers R, Easley K, Fausel Z, Robinson R. (2019) Functional Outcomes Following Microfragmented Adipose Tissue Versus Bone Marrow Aspirate Concentrate Injections for Symptomatic Knee Osteoarthritis. Stem Cells Transl Med. 8(11):1149-56.
-
Copcu HE, Oztan S. (2021) Not Stromal Vascular Fraction (SVF) or nanofat, but total stromal-cells (TOST): A new definition. Systemic Review of Mechanical Stromal-Cell Extraction Techniques. Tissue Eng Regen Med. 18(1):25-36.
-
Aronowitz JA, Lockhart RA, Hakakian CS. (2015) Mechanical versus enzymatic isolation of stromal vascular fraction cells from adipose tissue. SpringerPlus. 4:713.
-
Trivisonno A. (2019) Intraoperative Strategies for minimal manipulation of autologous adipose tissue for cell‚ and tissue-based therapies: concise review. Stem Cells Transl Med. 8(12):1265-71.
-
Tremolada C, Colombo V, Ventura C. (2016) Adipose Tissue and mesenchymal stem cells: state of the art and lipogems technology development. Curr Stem Cell Rep. 2(3):304-12.
-
Xu T, Yu X, Yang Q, Liu X, Fang J, et al. (2019) Autologous micro-fragmented adipose tissue as stem cell-based natural scaffold for cartilage defect repair. Cell Transplant. 28(12):1709-20.
-
Vezzani B. (2018) Higher pericyte content and secretory activity of microfragmented human adipose tissue compared to enzymatically derived stromal vascular fraction. Stem Cells Transl Med. 7(12):876-86.
-
Bouglé A, Rocheteau P, Hivelin M, Tremolada C, Manz J, et al. (2018) Micro-fragmented fat injection reduces sepsis induced acute inflammatory response in a mouse model. Br J Anaesth. 121(6):1249-59.
-
Mastrangelo AN, Haus BM, Vavken P, Palmer MP, Machan JT, et al. (2010) Murray Immature animals have higher cellular density in the healing anterior cruciate ligament than adolescent or adult animals. J Orthop Res. 28(8):1100-6.
-
Nicosia RF, Zorzi P, Ligresti G, Morishita A, Aplin AC. (2011) Paracrine regulation of angiogenesis by different cell types in the aorta ring model. Int J Dev Biol. 55(4-5):447-53
-
Bianchi F, Olivi E, Baldassarre M, Giannone FA, Laggetta M, et al. (2014) Lipogems, a new modality of fat tissue handling to enhance tissue repair in chronic hind limb ischemia. CellR4 2(6):e1289.
-
Filardo G, Tschon M, Perdisa F, Brogini S, Cavallo C, et al. (2021) Micro-fragmentation is a valid alternative to cell expansion and enzymatic digestion of adipose tissue for the treatment of knee osteoarthritis: A comparative preclinical study. Knee Surg Sports Traumatol Arthrosc. 30(3):773:81.
-
Casarotti GA, Chiodera P, Tremolada C. (2018) Menopause: new frontiers in the treatment of urogenital atrophy. Eur Rev Med Pharmacol Sci. 22(2):567-74.
-
Tremolada CM, Allegri M, Slevin. (2019) Mesenchymal stromal cells and micro fragmented adipose tissue: new horizons of effectiveness of lipogems. J Stem Cell Res Dev Ther. 5:017.
-
Tremolada C. (2017) Mesenchymal stem cells and regenerative medicine: how lipogems technology make them easy, safe and more effective to use. MOJ Biol Med. 2(2):223-26.
-
Raffaini M, Tremolada C. (2014) Micro fractured and purified adipose tissue graft (Lipogems®) can improve the orthognathic surgery outcomes both aesthetically and in postoperative healing. CellR4. 2(4):e1118.
-
Gobbi A, Dallo I, Rogers C. (2021) Two-year clinical outcomes of autologous microfragmented adipose tissue in elderly patients with knee osteoarthritis: a multi-centric, international study. Int Orthop. 45(5): 1179-88.
-
Hogaboom N, Malanga G, Cherian C, Dyson-Hudson T. (2021) A pilot study to evaluate micro-fragmented adipose tissue injection under ultrasound guidance for the treatment of refractory rotator cuff disease in wheelchair users with spinal cord injury. J Spinal Cord Med. 44(6):886-95.
-
Zeira O, Scaccia S, Pettinari L, Ghezzi E, Asiag N, et al. (2018) Intra-articular administration of autologous micro-fragmented adipose tissue in dogs with spontaneous osteoarthritis: Safety, feasibility, and clinical outcomes. Stem Cells Transl Med. 7(11):819-28.
-
Lavagnolo U, Veronese S, Negri S, Magnan B, Sbarbati A. (2021) Lipoaspirate processing for the treatment of knee osteoarthritis: a review of clinical evidences. Biomedicine & Pharmacother. 142:111997.
-
Heidari N, Borg TM, Olgiati S, Slevin M, Danovi A, et al. (2021) Microfragmented Adipose Tissue Injection (MFAT) May Be a Solution to the Rationing of Total Knee Replacement: A Prospective, Gender-Bias Mitigated, Reproducible Analysis at Two Years. Stem Cells Int. 2021:9921015.
-
Malanga GA, Chirichella PS, Hogaboom NS, capella T. (2021) Clinical evaluation of micro-fragmented adipose tissue as a treatment option for patients with meniscus tears with osteoarthritis: a prospective pilot study. Int Orthop. 45(2):473-80.
-
Zhou X, Zhang F, Wang D, Wang J, Wang C, et al. (2020) Micro fragmented adipose tissue promotes the matrix synthesis function of nucleus pulposus cells and regenerates degenerated intervertebral disc in a pig model. Cell Transplantat. 29:0963689720905798.
-
Malanga GA, Bemanian S. (2019) Microfragmented adipose injections in the treatment of knee osteoarthritis. J Clin Orthop Trauma. 10(1):46-48.
-
Robinson DM, Eng C, Mitchkash M, Tenforde AS, Borg-Stein J. (2020) Outcomes after Micronized Fat Adipose Transfer for Glenohumeral Joint Arthritis and Rotator Cuff Pathology: a Case Series of 18 Shoulders. MLTJ. 10(3):393-98.
-
Giori A, Tremolada C, Vailati R, Navone SE, Marfia G. (2015) Recovery of function in anal incontinence after micro-fragmented fat graft (Lipogems) injection: two years follow up of the first 5 cases. CellR4. 3(2):e1544.
-
Hudetz D, Boric I, Rod E, Jelec Z, Radic A, et al. (2017) The effect of intra-articular injection of autologous microfragmented fat tissue on proteoglycan synthesis in patients with knee osteoarthritis. Genes. 8(10):270.
-
Boric I, Hudetz D, Rod E, Jelec Z, Vrdoljak T, et al. (2019) A 24-month follow-up study of the effect of intra-articular injection of autologous microfragmented fat tissue on proteoglycan synthesis in patients with knee osteoarthritis. Genes. 10(12):1051.
-
Naldini G, Sturiale A, Fabiani B, Giani I, Menconi C. (2018) Micro-fragmented adipose tissue injection for the treatment of complex anal fistula: a pilot study accessing safety and feasibility. Tech Coloproctol. 22(2):107-13.
-
Casarotti G, Tremolada C. (2020) A new treatment of genito-urinary post-menopausal atrophy with autologous micro-fragmented fat tissue: a thirty-six months follow up case series. Eur Rev Med Pharmacol Sci. 24(13):7420-26.
-
Lonardi R, Leone N, Gennai S, Borsari GT, Covic T, et al. (2019) Autologous micro-fragmented adipose tissue for the treatment of diabetic foot minor amputations: a randomized controlled single-center clinical trial (MiFrAADiF). Stem Cell Res Ther. 10(1):223.
-
Hudetz D, Boric I, Rod E, Jelec Z, Kunovac B, et al. (2019) Early results of intra-articular micro-fragmented lipoaspirate treatment in patients with late stages knee osteoarthritis: A prospective study. Croat Med J. 60(3):227-36.
-
Laureti S, Gionchetti P, Capelli A, Vittori L, Contedini F, et al. (2020) Refractory complex Crohn's perianal fistulas: a role for autologous microfragmented adipose tissue injection. Inflamm Bowel Dis. 26(2):321-30.
-
Striano RD, Battista V, Bilboo N. (2017) Non-responding knee pain with osteoarthritis, meniscus and ligament tears treated with ultrasound guided autologous, micro-fragmented and minimally manipulated adipose tissue. Open Journal of Regenerative Medicine. 6(2):17-26.
-
Mikkelsen RK, Blond L, Holmich LR, Molgaard C, Troelsen A, et al. (2021) Treatment of osteoarthritis with autologous, micro-fragmented adipose tissue: a study protocol for a randomized controlled trial. Trials. 22(1):1-11.
-
Desando G, Bartolotti I, Martini L, Giavaresi G, Aldini NN, et al. (2019) Regenerative features of adipose tissue for osteoarthritis treatment in a rabbit model: enzymatic digestion versus mechanical disruption. Int J Mol Sci. 20(11):2636.
-
Alessandri G, Pessina A, Paroni R, Bercich L, Paino F, et al. (2021) Single-shot local injection of microfragmented fat tissue loaded with paclitaxel induces potent growth inhibition of hepatocellular carcinoma in nude mice. Cancers. 13(21):5505
-
Spees JL, Lee RH, Gregory CA. (2016) Mechanisms of mesenchymal stem/stromal cell function. Stem Cell Res Ther. 7(1):125.
-
Elahi FM, Farwell DG, Nolta JA, Anderson JD. (2020) Preclinical translation of exosomes derived from mesenchymal stem/stromal cells. Stem Cells. 38(1):15-21.
-
Tkach M, Thery C. (2016) Communication by extracellular vesicles: where we are and where we need to go. Cell. 164(6):1226–32
-
Yeo RWY, Lai RC, Zhang B, Tan SS, Yin Y, et al. (2013) Mesenchymal stem cell: an efficient mass producer of exosomes for drug delivery. Adv Drug Deliv Rev. 65(3):336–41.
-
Lin Y, Anderson JD, Rahnama LMA, Gu SV, Knowlton AA. (2020) Exosomes in disease and regeneration: biological functions, diagnostics, and beneficial effects. Am J Physiol Heart Circ Physiol. 319(6):H1162-180.
-
Oveili E, Vafaei S, Bazavar H, Eslami Y, Mamaghanizadeh E, et al. (2023) The potential use of mesenchymal stem cells-derived exosomes as microRNAs delivery systems in different diseases. Cell Commun Signal. 21(1):20.
-
Martone WJ, Nichols RL. (2001) Recognition, prevention, surveillance, and management of surgical site infections: introduction to the problem and symposium overview. Clin Infect Dis. 33 suppl 2:S67-8
-
Alcayaga-Miranda F, Cuenca J, Khoury M. (2017) Antimicrobial activity of mesenchymal stem cells: current status and new perspectives of antimicrobial peptide-based therapies. Front Immunol. 8:339.
-
Fleischmann C, Scherag A, Adhikari NK, Hartog CS, Tsaganos T, et al. (2016) Assessment of Global Incidence and Mortality of Hospital-treated Sepsis. Current Estimates and Limitations. Am J Respir Crit Care Med. 193(3):259-72.
-
Angus DC, van der Poll T. (2013) Severe sepsis and septic shock. N Engl J Med. 369:840-51
-
Singer M, Deutschman CS, Seymour CW, Shankar-Hari M, Annane D, et al. (2016) The Third International Consensus Definitions for Sepsis and Septic Shock (Sepsis-5283). JAMA. 315(8):801–10.
-
Martínez ML, Ferrer R, Torrents E, Guillamat-Prats R, Gomà G, et al. (2017) Impact of source control in patients with severe sepsis and septic shock. Crit Care Med. 45(1):11-19
-
Levy MM, Fink MP, Marshall JC, Abraham E, Angus D, et al. (2003) 2001 SCCM/ESICM/ACCP/ATS/SIS inter- national sepsis definitions conference. Crit Care Med. 31(4):1250-56.
-
https://www.who.int/news/item/08-09-2020-who-calls-for-global-action-on-sepsis---cause-of-1-in-5-deaths-worldwide
-
Alessandri G, Cocce V, Pastorino F, Paroni R, Cas MD, et al. (2019) Microfragmented human fat tissue is a natural scaffold for drug delivery: Potential application in cancer chemotherapy. J Control Release. 302:2-18
-
Salagean S, Nechifor-Boila A, Bajwa N, Pastorello Y, Slevin M. (2023) Micro-fragmented adipose tissue as a natural scaffold for targeted drug delivery in brain cancer. Int J Mol Sci. 24(14):11530
-
Zeira O, Ghezzi E, Pettinari L, Re V, Lupi DM, et al. (2021) Case Report: Microfragmented Adipose Tissue Drug Delivery in Canine Mesothelioma: A Case Report on Safety, Feasibility, and Clinical Findings. Front Vet Sci. 7:585427.