Mesenchymal Stem Cell Therapy for Chronic Wound Healing
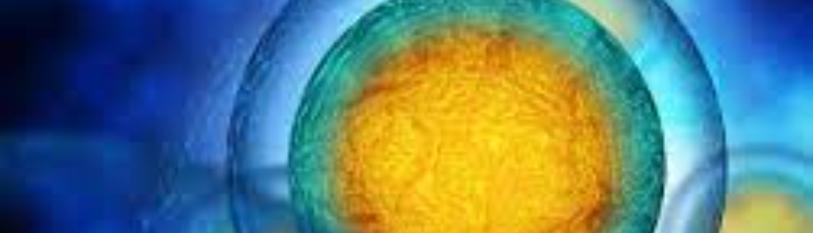
Samantha Spence and Vincent S. Gallicchio*
Department of Biological Sciences, College of Science, Clemson University, Clemson, SC 29636
*Corresponding author: Vincent S. Gallicchio, Department of Biological Sciences, College of Science, Clemson University, Clemson, SC 29636
Citation: Spence S, Gallicchio VS. (2022)Mesenchymal Stem Cell Therapy for Chronic Wound Healing. J Stem Cell Res. 3(1):1-13.
Received: December 07, 2021 | Published: January 03, 2022
Copyright© 2022 genesis pub by Spence S, et al. CC BY-NC-ND 4.0 DEED. This is an open-access article distributed under the terms of the Creative Commons Attribution-Non Commercial-No Derivatives 4.0 International License., This allows others distribute, remix, tweak, and build upon the work, even commercially, as long as they credit the authors for the original creation.
DOI: https://doi.org/10.52793/JSCR.2022.3(1)-25
Abstract
Chronic or non-healing skin wounds present an ongoing challenge in advanced wound care and the current wound healing technologies remain insufficient. Recently, stem cell therapy has emerged as a promising new approach for chronic wound healing, with mesenchymal stem cells (MSCs) being of the most interest. MSCs have proven to be an attractive cell type for cell-based therapy due to their ease of isolation, vast differentiation potential, and immunomodulatory effects during transplantation. Furthermore, MSCs were determined to play an innate role in the wound healing process making them an obvious candidate for treatment of chronic wounds. When introduced into the wound bed, MSCs have been shown to promote fibroblast migration, stimulate extracellular matrix (ECM) deposition, facilitate wound closure, initiate re-epithelialization, enhance angiogenesis, and mitigate inflammation in preclinical animal models. The efficacy and safety of MSC application for treatment of chronic wounds was further confirmed by several clinical studies involving human subjects which yielded similar positive results with no adverse side effects. However, while MSCs appear to be a promising resource for chronic wound care, more research is required to determine the optimal cell source and route of delivery before this technology can be applied in clinical medicine.
Keywords
Chronic wound; Wound healing; Mesenchymal stem cell; Cell therapy
Introduction
Despite advances in medical and surgical wound care, treatment of chronic cutaneous wounds remains a significant biomedical burden. Wound healing is a complex, multifactorial process which occurs in three distinct, yet overlapping phases: inflammation, cell proliferation, and remodeling. The inflammatory phase immediately follows wounding and initially serves to stop bleeding in the wound bed via platelet aggregation and clear the wound of dead cells, debris, and residual extracellular matrix (ECM) via immune cell recruitment. The proliferative phase involves fibroblast migration into the wound bed which stimulates the deposition of new ECM as well as neovascularization of the skin through the upregulation of angiogenic factors. The remodeling phase is the last phase of the wound healing process and is characterized by the deposition and degradation of various collagens to increase the strength of the new skin [1,2] (Figure 1).
Figure 1: Phases of physiological wound healing. Inflammatory phase: hemostasis of the wounded area and acute inflammation. Proliferative phase: increase in cell migration, synthesis of extracellular matrix components, angiogenesis, and re-epithelialization. Remodeling phase: extracellular matrix remodeling [3].
A chronic or non-healing wound is one which does not progress normally through the wound healing process and is unable to regain anatomic and functional integrity. Chronic wounds can be caused by various pathophysiological conditions including malnutrition, immunosuppression, diabetes mellitus, and vascular compromise [4]. Currently biological techniques for advanced wound care typically focus on modification of controllable causative factors using antibiotics, pressure relief, or compression garments for venous insufficiency. However, it has been found that these treatments are able to achieve only a 50% healing rate, the results of which are often temporary [1]. Therefore, there is still a critical need for new methods of enhancing the wound healing process to optimize patient outcomes. Recently, stem cell therapy has emerged as a promising new approach for enhancing tissue repair and regeneration following injury.
Mesenchymal stem cells (MSCs) are multipotent progenitor cells which have traditionally been identified for their ability to leave their niche to home towards sites of injury and engraft with foreign tissues, making them attractive candidates for a variety of cell-based therapies [5]. Easily obtainable from several tissues, including bone marrow, adipose tissue, and the umbilical cord, MSCs are also characterized by their ability to self-renew and differentiate into multiple tissue-forming cell lineages. More recently, endogenous MSCs have been determined to play an innate role in the wound healing process and are therefore of particular interest for application in wound healing therapies [2,6]. Through several preclinical and clinical trials, MSCs have been found to accelerate cutaneous wound healing by promoting regeneration and remodeling of the skin through cellular recruitment and direct differentiation, neovascularization through the secretion and upregulation of angiogenic factors, and immunomodulation through the activation or suppression of immune cells [7-9].
Effects of Mesenchymal Stem Cells (MSC) on the Wound Healing Process
Wound healing is an intricate process requiring the well-orchestrated integration of the biological and molecular events of cell migration and proliferation, ECM deposition and remodeling, wound contraction, angiogenesis, and immunomodulation. MSCs have been found to play a vital role in promoting each of these events (Figure 2).
Fibroblast Migration
Fibroblasts are crucial players in the proliferative phase of normal wound healing and are involved in several key processes such as creating new ECM and collagen structures to support the other cells associated with effective wound healing as well as contracting the wound. In abnormal wound repair, dermal fibroblast responses to injury are impaired and dysregulated, representing a major obstacle to successful healing. However, several preclinical studies suggest that MSCs can stimulate the proliferative phase of healing in chronic wounds through the promotion of fibroblast proliferation and accelerated migration in scratch assays [10-12]. Additionally, a study by Andria N. Smith et. al., demonstrated bone marrow-derived mesenchymal stem cells (BM-MSCs) secrete a soluble chemoattractant for dermal fibroblasts, supporting the directional migration of these cells towards the MSC-seeded wound bed. Smith’s group also found that MSCs induce dermal fibroblasts to regulate expression of genes involved in ECM homeostasis and intercellular adhesion, both of which play critical roles in wound healing [8].
Collagen and Extracellular Matrix (ECM) Deposition
Once localized in the wound bed, fibroblasts are responsible for the synthesis and deposition of collagen, proteoglycans, and other components that comprise ECM. Collagen is of particular importance in wound healing because it serves as a scaffold in the connective tissue ECM to maintain dermal structure. The collagen types I and III are the main collagen types of healthy skin and the ratio of these two types can be used to determine the progress of wound repair, with collagen III being more favorable for scarless healing. A study by Lingying Lui et. al., demonstrated that severe burn wounds treated with human umbilical cord-derived mesenchymal stem cells (UC-MSCs) showed increased accumulation of collagen types I and III after transplantation into a rat burn model [2]. Furthermore, the application of adipose-derived mesenchymal stem cells (A-MSCs) in a murine incisional model showed an increased ratio of collagen III to collagen I in vivo which mitigated scar formation [13]. MSCs have also been found to suppress the degradation of the collagenous matrix and preserve the structural integrity of the ECM through the downregulation of matrix metalloproteinase-1 (MMP-1), a type of collagenase which primarily breaks down collagen types I, II, and III [8, 11]. Lastly, Young Keul Jeon, et. al., found that, along with the regulation of collage synthesis and degradation, MSCs also promote the synthesis of elastin which is responsible for providing tissue resiliency and fibronectin which is primarily involved in cell-adhesive interactions [11].
Wound Contraction
Following connective tissue matrix formation by collagen fibers, fibroblasts differentiate into myofibroblasts to stimulate wound contraction. Both fibroblasts and myofibroblasts play a critical role in wound contraction by generating traction and contractile forces, respectively, to pull the wound edges toward the wound center [14]. As MSCs have been previously proven to enhance fibroblast migration and proliferation, their introduction into the wound bed should in theory accelerate the time to wound closure. This idea has been verified in studies involving scratch wound assays and animal excisional wound models which showed significantly enhanced wound closure rates when treated with MSCs as compared to controlled treatments [1,10,15,16].
Epithelialization
The re-epithelialization process involves the formation of new epithelium and skin appendages by activating the proliferation and differentiation of keratinocytes and their subsequent migration from the wound edge over the denuded area. While MSCs do have the ability to spontaneously differentiate into keratinocytes in the wound tissue [15,16], their impacts on epithelialization in wound healing remain largely paracrine. MSCs have been found to enhance keratinocyte migration and proliferation through the secretion of various pro-migratory factors including transforming growth factor beta (TGF-β) [4,10]. In turn, skin models treated with MSCs have been shown to give rise to a multi-layered, well-differentiated epidermis comparable to the human epidermis [17]. A study by Gaoxing Luo et. al., further demonstrated that MSCs increased the thickness of the regenerated epidermis, increased the dermal ridges and number of cells in regenerated skin, and produced granulation tissue with more regular alignment of fibers in immunodeficient murine models. The same study also showed that wounds treated with MSCs additionally developed hair follicles, sweat glands, and other normal skin appendages [16]. This suggests that the administration of MSCs may be able to not only accelerate wound healing, but also enhance wound healing quality and the physiological functioning of the regenerated skin.
Angiogenesis
In wound healing, the formation of new blood vessels is necessary to sustain the newly formed granulation tissue and the survival of keratinocytes in the wound bed. Neovascularization is accomplished by MSCs through both direct differentiation into endothelial cell lineages and upregulation of angiogenic factors through paracrine signaling. A study by Ying Cao, et. al., demonstrated that A-MSCs can differentiate in response to local cues into endothelial cells that contribute to neovascular structures in hindlimb ischemia models [18]. However, MSCs primarily support endothelial cell tube formation through the secretion and upregulation of vascular endothelial growth factor (VEGF) and angiopoietin-1 (Ang-1). VEGF is the most effective and specific growth factor in regulating angiogenesis which stimulates endothelial cell proliferation, migration, and organization into tubules. Ang-1 mediates vascular maturation and helps to maintain vessel integrity [5]. Markedly high levels of VEGF and Ang-1 were found in murine wound models treated with MSCs which resulted in increased density of neovessels, enhanced complexity of vascular structures, and overall improvement in cutaneous wound microcirculation [1,15,19].
Immunomodulation
A major problem in chronic wounding is unmitigated inflammation. While invasion of the wound bed by immune cells during the inflammatory phase of healing is crucial for initial wound cleaning, a continuous state of inflammation in the wound can create a cascade that perpetuates a nonhealing state. Therefore, it is beneficial to decrease the quantity of infiltrated inflammatory cells so that wound healing can progress. MSCs have been shown to attenuate the inflammation of locally burned skin by decreasing neutrophil and macrophage infiltration and pro-inflammatory cytokine production while increasing the production of anti-inflammatory cytokines [19]. Eunkyung Chung, et. al., also demonstrated that MSCs can transform pro-inflammatory M1 macrophages into anti-inflammatory M2 macrophages which facilitate resolution of inflammation, promotion of tissue remodeling, and elimination of tissue debris [20]. Furthermore, MSCs have been found to downregulate the expression of intracellular adhesion molecule 1 (ICAM1), which is known to mediate the binding of leukocytes to dermal fibroblasts, therefore indicating a role in inflammation [8,21]. Lastly, preclinical studies exploring the effect of UC-MSCs on regulation of oxidative stress found that MSC conditioned media can inhibit superoxide radical production to basal levels and promote the synthesis of superoxide dismutase to optimize the healing environment and promote wound closure [11,22].
Current Clinical Applications
Consistent with preclinical studies, several clinical trials have demonstrated improved wound healing outcomes following the application of MSCs in chronic wounds with no documented adverse effects. In a recent review of completed and ongoing clinical studies, Yi-Zhou Huang et. al., outlined the methodology, status, and outcome of all reported human case and clinical studies in which MSCs were used to treat non-healing wounds [23] (Figure 2).
Figure 2: Enhanced cutaneous wound healing by mesenchymal stem cells. Largely via paracrine signaling, MSCs can control cell migration as well as up- and downregulate certain proteins and transcription factors to promote wound contraction, ECM remodeling, epithelialization, and angiogenesis while decreasing inflammation [23].
Across these studies, MSCs have been found to accelerate time to wound closure, decrease wound size, improve dermal remodeling, increase neovascularization, and reduce inflammation in non-healing wounds. For example, a study involving local implantation of MSCs in a hydrogel sheet to diabetic foot ulcers showed a significant decrease in time to wound closure as compared to control groups. 82% of wounds dressed in A-MSC sheets achieved complete wound closure after 12 weeks, while only 53% in the control group achieved complete closure. Furthermore, the median times to complete closure were 28.5 days and 63.0 days for the treatment group and the control group, respectively [24]. Similarly, another study by Vincent Falanga et. al., demonstrated that application of MSCs to chronic wound beds using a fibrin polymer spray system resulted in accelerated wound healing with a strong direct correlation between number of cells applied and subsequent decrease in wound size. Furthermore, they confirmed that the applied cells were able to establish themselves in the wound bed and promote the migration and differentiation of other supportive cells, such as fibroblasts and endothelial cells. Interestingly, new elastic fibers were also found to be deposited in the dermis of treated full-thickness wounds, which does not normally occur in healing wounds or scars, suggesting that MSCs may have the power to not only support wound closure, but also recover skin structure and function [25]. Dermal rebuilding was similarly observed in a study involving autologous BM-MSCs applied topically to chronic wounds in human subjects. Significant thickening of the skin was noted in all patients as well as the laying down of collagen fibers and the presence of ground substance. Improved microcirculation was also seen resulting from increased vascularity of the wound bed and surrounding area [26]. Substantial angiogenesis was also observed in a study of chronic radiation-induced skin lesions treated with BM-MSCs as well as a significant reduction in inflammation, both of which allowed for more efficient wound healing and improved skin quality [27]. In each of these studies, no serious adverse events or were reported, and no wound recurrence or tumorigenicity was observed in the months following treatment. Therefore, it can be concluded that MSC-based therapy represents both a safe and effective treatment options for chronic wounds. However, more research regarding cell source, dose, timing, and route of administration is required before this technology can be confidently implemented into modern wound therapy (Table 1).
Wound |
Investigator |
Source |
Delivery |
Phase |
Outcome |
LEU
|
Lafosse et al. (2015) |
AT |
Local implantation in a collagen matrix |
CR |
Improved dermal angiogenesis and remodeling |
Moon et al. (2019) |
AT |
Local implantation in a hydrogel sheet |
RCT |
Higher rate of complete wound closure; reduced Kaplan-Meier median time to complete closure |
|
Falanga et al. (2007) |
BM |
Local implantation in a fibrin gel |
CR |
Wounds healed within 20 weeks |
|
Dash et al. (2009) |
BM |
Local delivery |
RCT |
Reduced ulcer size |
|
Lu et al. (2011) |
BM |
Local injection |
RCT |
Promoted foot ulcer healing |
|
Kirana et al. (2012) |
BM |
Intramuscular or intraarterial injection in lower limb |
RCT |
Improve microcirculation; support wound healing in diabetic foot patients without option of revascularization |
|
Benítez-Arvízu et al. (2015) |
BM |
Local implantation in PRP |
CR |
The wound healed; no complications in 1-year follow-up |
|
Maksimova et al. (2017) |
BM |
Topical application of cell suspension |
CR |
Accelerated reepithelialization; all wounds were closed successfully |
|
Hashemi et al. (2019) |
Wharton’s jelly |
Local implantation with acellular amniotic membrane |
CR |
Significantly decreased wound-healing time and wound size |
|
Qin et al. (2016) |
Umbilical cord |
Endovascular infusion and injection around ulcer |
RCT |
No serious complications; increased neovessels, complete or gradual ulcer healing |
|
Wu et al. (2017) |
Placenta |
Deep intramuscular injection into the calf of leg with index ulcer |
Phase 1 |
No serious adverse events; preliminary evidence of ulcer healing within 3 months |
|
Zeng et al. (2017) |
Placenta |
Topical application in an alginate gel |
CR |
The ulcer was almost healed; no complications; no recurrence in the follow-up of 6 months |
|
Radiation burns
|
Lataillade et al. (2007) |
BM |
Local injection |
CR |
Decreased wound size; almost complete healing 1-month posttreatment |
Bey et al. (2010) |
BM |
Local injection |
CR |
No recurrence of radiation inflammatory waves in 8-month follow-up |
|
Portas et al. (2016) |
BM |
Local implantation in a fibrin matrix |
CR |
Reduced inflammation, improved vasculature and skin quality |
|
Pressure sores |
Yoshikawa et al. (2008) |
BM |
Local implantation in a collagen sponge |
CR |
The ulcer almost healed in 9 of 11 pressure sore patients; the ulcer did not recur for at least 1-year in seven patients |
Table 1: Clinical Studies of Mesenchymal Stem Cell-Based Therapy for Chronic Wounds in the Literature. Presents an outline of all clinical studies reported in literature to date, organized by wound type, investigators, cell source, delivery method, phase of study, and outcome [23].
Future Questions
Cell source
MSCs can be isolated from various tissues and exhibit different potential for clinical applications according to their origin. While bone marrow (BM) is the mostly widely studied and accepted source of multipotent MSCs, the invasive procedure necessary to harvest these cells, the risks of complications, and the age-dependent decline of self-renewal capacity of MSCs has led to a search for alternate sources. Adipose tissue (AT) has been recognized as another alternative source of MSCs that can be obtained in larger quantities by a less invasive method via liposuction and grown easily under standard tissue culture conditions. More recently, placenta-derived MSCs (P-MSCs) and umbilical cord-derived MSCs (UC-MSCs) have become of interest in cell therapy due to their minimally invasive method of isolation from the placenta and umbilical cord blood (UCB), respectively, which poses no harm to the mother or the infant. However, controversy and complications surrounding these methods still exist due to the inevitable allogeneity of the cell source and the lower isolation success rate [28].
There are appreciable differences in the biological properties of MSCs derived from these different tissues, ranging from differentiation potential to immunomodulatory ability. Several comparative analyses have been conducted to compare the cell morphology, immunophenotype, gene expression profile, proliferation potential, differentiation potential, and immunomodulatory MSCs isolated from BM, AT, UCB, and placenta. Studies by June Seok Heo et. al. [29] and Susanne Kern et. al. [28], found no significant differences in morphology, clonogenic efficiency, or immunophenotype of cells isolated from these different sources but found considerable differences in terms of proliferative rates and differentiation potential. While P-MSCs were noted to have the fastest growth rate, both BM-MSCs and A-MSCs were determined to have greater multilineage differentiation potential than either P-MSCs or UCB-MSCs when cultured in vitro. BM- and A-MSCs were also found to have similar gene expression profiles and were the only two cell types which significantly inhibited T-cell proliferation. Therefore, due to their regenerative and immunosuppressive properties, MSCs derived from adult tissues represent a preferred cell type in the field of regenerative medicine and appear to hold the most promise in wound treatment. However, the role of specific cell subpopulations and their individual wound-healing potentials remain unknown. Future studies are recommended to determine the influence of tissue origin of MSCs on their wound-healing ability to identify which cell population possesses the most beneficial therapeutic effect.
Delivery Method
Several delivery methods have been used to introduce MSCs into wound beds, however it is yet to be determined which technique is the safest and most efficient. One of the first delivery methods to be studied was intravenous (IV) injection of MSCs. However, even though stem cells are known to home to sites of injury, IV injected MSCs have been shown to remain trapped within pulmonary microcirculation for the first 3 days post-injection [30]. Local delivery of stem cells, via local injection, topical application, or incorporation into a three-dimensional scaffold, has the potential to circumvent this issue. Traditionally, most studies have used intradermal injection of MSCs into or around the wound area. Although this method has been shown to improve wound healing, the ultimate therapeutic potential of this technique seems to be limited by poor engraftment efficiency and cell retention in the wound bed [31]. Therefore, alternative methods that increase delivery and survival of MSCs at the wound site are desirable. Topical application, via fibrin spray, has been shown to sufficiently stimulate healing and closure of full-thickness chronic wounds, especially when paired with a biologic wound dressing [25, 26, 32]. However, topical application is limited by delivery of nonprotected cells into the wound environment as well as poor control of cell density and spacing [33]. More recently, stem cell-seeded 3D scaffolds have emerged as a promising delivery technique to circumvent these issues and establish a functional niche in which these cells can survive and proliferate. 3D scaffolds can be composed of hydrogel, collagen, or a variety of other natural or synthetic materials and provide cells with a matrix allowing for attachment and protection from the hostile wound environment. Several studies have deemed biomaterial scaffolds superior to other forms of local delivery in terms of localizing cells within wounds, enhancing stem cell characteristics, preserving cell-matrix interactions, promoting angiogenesis, and subsequently accelerating wound healing [34, 35]. For example, stem cells delivered in a hydrogel matrix remained viable longer and demonstrated enhanced engraftment efficiency as compared to MSCs introduced via local injection. Wounds treated with MSC-seeded hydrogels additionally showed significantly accelerated healing, return of skin appendages, and enhanced angiogenesis [29]. Furthermore, local implantation of MSCs in a collagen matrix was found to promote neovascularization throughout the scaffold, allowing for the transport of nutrients and reparative cell types across the wound bed which is necessary for proper healing and closure [6]. Beyond basic mechanical protection, Dominik Duscher et. al., found that collagen bioscaffolds can quench free radicals within ischemic wounds, thereby decreasing oxidative stress and subsequent inflammation and increasing graft cell longevity [33]. While 3D stem cell-seeded bioscaffolds seem to hold the most promise in treatment of chronic wounds, more studies are required to determine the best scaffold composition to optimize wound healing results before this technology can be applied in clinical medicine.
Discussion
The use of MSCs for the treatment of chronic wounds has proven to be feasible, effective, and safe, as demonstrated through numerous preclinical and clinical trials. MSCs have been found to stimulate the wound healing process in chronic wounds through several biological and molecular mechanisms. One of the primary roles of MSCs in wound healing is promoting the directional migration of fibroblast cells to the site of injury where they can localize in the wound bed. Once localized, these fibroblasts work to facilitate wound closure and synthesize the necessary components of the ECM, such as collagen. Additionally, MSCs have been proven to downregulate MMP-1, a type of collagenase primarily responsible for ECM degradation. In this way, MSCs work to preserve the ECM and maintain dermal structure. Wounds treated with MSCs have also been shown to have increased levels of elastin which provides the tissue with resiliency and is not typically seen in normal wound healing. MSCs also play a role in the re-epithelialization process by activating the proliferation, differentiation, and migration of keratinocytes which in turn supports the formation of a multi-layered and well-differentiated epidermis. MSCs are also believed to stimulate the development of new skin appendages such as hair follicles and sweat glands which suggests these stem cells have the power to not only accelerate wound healing but also improve wound healing quality. Furthermore, the application of MSCs to chronic wounds supports angiogenesis by upregulating VEGF and Ang-1 and increasing the density of microvessels throughout the wound bed. This allows for the transport of nutrients and oxygen to the developing cells, enhancing their longevity. Lastly, MSCs help to modulate the hostile wound environment and in turn support proper healing by mitigating inflammation at the site of injury. MSCs decrease infiltration of inflammatory cells and pro-inflammatory cytokines and initiate the polarization of M1 macrophages to anti-inflammatory M2 macrophages. MSCs also downregulate ICAM1, a protein involved in inflammation, and upregulate superoxide dismutase, an enzyme which breaks down harmful superoxide radicals. In this way, MSCs have proven to support wound healing by optimizing the healing environment and promoting efficient wound closure.
Several clinical trials have yielded the same positive results when MSCs were applied to chronic or non-healing wounds in human subjects. Furthermore, no adverse side effects have been observed which confirms the safety and feasibility of this technology for human application. However, further research must be done to determine the best cell source and route of delivery before this technique can be utilized in modern wound care. MSCs can be isolated from various tissue types including BM, AT, UCB, and placenta and these cells demonstrate unique properties and potentials depending on the source. Several comparative studies have pointed towards A-MMSCs as holding the most promise for cell therapy due to their abundance and ease of isolation as well as their regenerative and immunomodulatory properties. The next question that must be addressed concerns the route of delivery of these stem cells into the wound bed. MSCs can be locally delivered to the wound bed via injection, topical application, or incorporation into a 3D scaffold to circumvent issues related to low engraftment efficiency with IV injection. Of these three local delivery methods, seeding of the MSCs into a biomaterial scaffold has appeared to hold the most promise as it allows for the localization of the cells into the wound bed and provides the donor cells with protection and structure. With further research into these areas, the application of MSCs to chronic or non-healing wounds could represent a major development in advanced wound care.
References
- Wu Y, Chen L, Scott PG, Tredget EE. (2007) Mesenchymal stem cells enhance wound healing through differentiation and angiogenesis. Stem Cells. 25(10):2648-69.
- Nuschke A. (2013). Activity of mesenchymal stem cells in therapies for chronic skin wound healing. Organogenesis, 10(1):29-37.
- Gushiken LF, Beserra FP, Bastos JK, Jackson CJ, Pellizzon CH. (2021) Cutaneous wound healing: An update from physiopathology to current therapies. Life. 11(7):665.
- Lee DE, Ayoub N, Agrawal DK. (2016) Mesenchymal stem cells and cutaneous wound healing: Novel methods to increase cell delivery and therapeutic efficacy. Stem Cell Res Therapy. 7(1).
- Li H, Fu X, Ouyang Y, Cai C, Wang J, et al. (2006) Adult bone-marrow-derived mesenchymal stem cells contribute to wound healing of skin appendages. Cell and Tissue Res. 326(3):725-36.
- Jackson WM, Nesti LJ, Tuan RS. (2011) Concise review: Clinical translation of wound healing therapies based on mesenchymal stem cells. STEM CELLS Transl Med. 1(1):44-50.
- Isakson M, de Blacam C, Whelan D, McArdle A, Clover AJ. (2015) Mesenchymal stem cells and cutaneous wound healing: Current evidence and future potential. Stem Cells Int. 2015:1-12.
- Smith AN, Willis E, Chan VT, Muffley LA, Isik FF, et al. (2010) Mesenchymal stem cells induce dermal fibroblast responses to injury. Experimental Cell Res. 316(1):48-54.
- DiMarino AM, Caplan AI, Bonfield TL. (2013) Mesenchymal stem cells in tissue repair. Frontiers in Immunology. 4.
- Walter MNM, Wright KT, Fuller HR, MacNeil S, Johnson WEB. (2010) Mesenchymal stem cell-conditioned medium accelerates skin wound healing: An in vitro study of fibroblast and keratinocyte scratch assays. Experimental Cell Res. 316(7):1271-81.
- Jeon YK, Jang YH, Yoo DR, Kim SN, Lee SK, et al. (2010). Mesenchymal stem cells' interaction with skin: Wound-healing effect on fibroblast cells and skin tissue. Wound Repair and Regen. 18(6):655-61.
- Arno AI, Amini-Nik S, Blit PH, Al-Shehab M, Belo C, et al. (2014) Human Wharton’s jelly mesenchymal stem cells promote skin wound healing through paracrine signaling. Stem Cell Research & Ther. 5(1):28.
- Wang L, Hu L, Zhou X, Xiong Z, Zhang C, et al. (2017) Exosomes secreted by human adipose mesenchymal stem cells promote scarless cutaneous repair by regulating extracellular matrix remodeling. Scientific Reports. 7(1).
- Li B, Wang JHC. (2011) Fibroblasts and myofibroblasts in wound healing: Force generation and measurement. J Tissue Viability, 20(4):108-20.
- Nie C, Yang D, Xu J, Si Z, Jin X, et al. (2011) Locally administered adipose-derived stem cells accelerate wound healing through differentiation and vasculogenesis. Cell Transplant. 20(2): 205-16.
- Luo G, Cheng W, He W, Wang X, Tan J, et al. (2010) Promotion of cutaneous wound healing by local application of mesenchymal stem cells derived from human umbilical cord blood. Wound Repair and Regen. 18(5):506-13.
- Ojeh NO, Navsaria HA. (2013) An in vitro skin model to study the effect of mesenchymal stem cells in wound healing and epidermal regeneration. J Biomedical Materials Res. 102(8):2785-92.
- Cao Y, Sun Z, Liao L, Meng Y, Han Q, et al. (2005) Human adipose tissue-derived stem cells differentiate into endothelial cells in vitro and improve postnatal neovascularization in vivo. Biochem Biophys Res Commun. 332(2):370-79.
- Liu L, Yu Y, Hou Y, Chai J, Duan H, et al. (2014). Human umbilical cord mesenchymal stem cells transplantation promotes cutaneous wound healing of severe burned rats. PLoS ONE. 9(2):e88348.
- Chung E, Son Y. (2014) Crosstalk between mesenchymal stem cells and macrophages in tissue repair. J Tissue Eng Regen Med. 11(6):431-38.
- Couture P, Paradis-Massie J, Oualha N, Thibault G. (2009). Adhesion and transcellular migration of neutrophils and B lymphocytes on fibroblasts. Exp Cell Res. 315(13):2192-206.
- Torrente D, Avila MF, Cabezas R, Morales L, Gonzalez J, et al. (2013). Paracrine factors of human mesenchymal stem cells increase wound closure and reduce reactive oxygen species production in a traumatic brain injury in vitro model. Hum Exp Toxicol. 33(7): 673-84.
- Huang YZ, Gou M, Da LC, Zhang WQ, Xie HQ. (2020) Mesenchymal stem cells for chronic wound healing: Current status of preclinical and clinical studies. Tissue Engineering Reviews. 26(6):555-70.
- Moon KC, Suh HS, Kim KB, Han SK, Young KW, et al. (2019) Potential of allogeneic adipose-derived stem cell – hydrogel complex for treating diabetic foot ulcers. Diabetes. 68(4):837-46.
- Falanga V, Iwamoto S, Chartier M, Yufit T, Butmarc J, et al. (2007) Autologous bone marrow–derived cultured mesenchymal stem cells delivered in a fibrin spray accelerate healing in murine and human cutaneous wounds. Tissue Engin. 13(6):1299-1312.
- Badiavas EV. (2003) Treatment of chronic wounds with bone marrow–derived cells. Archives of Dermatol. 139(4):510.
- Portas M, Mansilla E, Drago H, Dubner D, Radl A, et al. (2016) Use of human cadaveric mesenchymal stem cells for cell therapy of a chronic radiation-induced skin lesion: A case report. Radiat Prot Dosim. 171(1):99-106.
- Kern S, Eichler H, Stoeve J, Klüter H, Bieback K. (2006) Comparative analysis of mesenchymal stem cells from bone marrow, umbilical cord blood, or adipose tissue. Stem Cells. 24(5):1294-1301.
- Heo JS, Choi Y, Kim HS, Kim H. (2015) Comparison of molecular profiles of human mesenchymal stem cells derived from bone marrow, umbilical cord blood, placenta and adipose tissue. Int J Mol Med. 37(1):115-125.
- Rustad KC, Wong VW, Sorkin M, Glotzbach JP, Major MR, et al. (2012) Enhancement of mesenchymal stem cell angiogenic capacity and Stemness by a biomimetic hydrogel scaffold. Biomaterials. 33(1):80-90.
- Wagner J, Kean T, Young R, Dennis JE, Caplan AI. (2009) Optimizing mesenchymal stem cell-based therapeutics. Curr Opin Biotechnol. 20(5):531-36.
- Dash NR, Dash SN, Routray P, Mohapatra S, Mohapatra PC. (2009) Targeting nonhealing ulcers of lower extremity in human through autologous bone marrow-derived mesenchymal stem cells. Rejuvenation Res. 12(5):359-66.
- Duscher D, Barrera J, Wong VW, Maan ZN, Whittam AJ, et al. (2015). Stem cells in wound healing: The future of regenerative medicine? A mini-review. Gerontology. 62(2):216-25.
- Sorice S, Rustad KC. Li AY, Gurtner GC. (2016) The role of stem cell therapeutics in wound healing: Current understanding and future directions. Plast Reconstr Surg. 138:31S-41S.
- Yoshikawa T, Mitsuno H, Nonaka I, Sen Y, Kawanishi K, et al. (2008) Wound therapy by Marrow Mesenchymal Cell Transplantation. Plast Reconstr Surg. 121(3):860-77.