Mesenchymal Stem Cell-Based Therapy for Advanced Colorectal Cancer: Potential Mechanisms of Therapy and Associated Risks
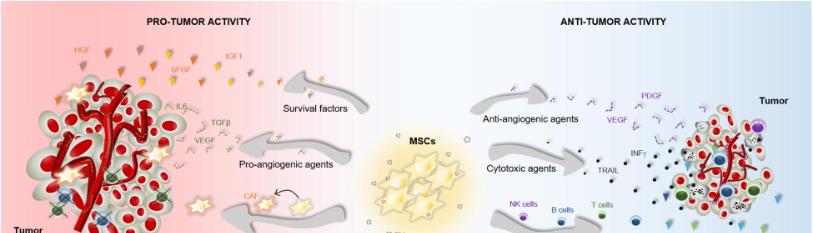
Annie Hilland and Vincent S Gallicchio*
Department of Biological Sciences, College of Science, Clemson University, Clemson, South Carolina, USA
*Corresponding author: Vincent S Gallicchio, Department of Biological Sciences, College of Science, Clemson University, Clemson, South Carolina, USA
Citation: Hill A, Gallicchio VS. (2021) Mesenchymal Stem Cell-Based Therapy for Advanced Colorectal Cancer: Potential Mechanisms of Therapy and Associated Risks. J Stem Cell Res. 2(2):1-11.
Received: April 27, 2021 | Published: May 25, 2021
Copyright© 2021 genesis pub by Hill A, et al. CC BY NC-ND 4.0 DEED. This is an open-access article distributed under the terms of the Creative Commons Attribution-NonCommercial-No Derivatives 4.0 International License., This allows others distribute, remix, tweak, and build upon the work, even commercially, as long as they credit the authors for the original creation.
DOI: https://doi.org/10.52793/jscr.2021.2(2)-S4
Abstract
Despite recent advances in diagnosis and treatment, colorectal cancer (CRC) remains one of the leading causes of cancer-related deaths due to therapy resistance. Traditional treatment methods for colon cancer include surgery, chemotherapy, and radiation therapy. However, due to the unspecific target of these strategies and the presence of therapy-resistant colon cancer stem cells in the tumor microenvironment, nearly 50% of patients exhibit tumor recurrence. Therefore, there is an urgent need for alternative methods, and targeted cell-based therapies are emerging as promising agents for the treatment of heterogeneous malignancies. In particular, mesenchymal stem cells (MSCs) have been widely investigated for their therapeutic potential in regenerative medicine due to unique biological features, including self-regeneration and differentiation potential, tumor homing capacity, and immunomodulatory and paracrine functions. However, the exact role of MSCs in cancer treatment remains controversial as they have been shown to be involved in tumor progression and inhibition at the preclinical level. This review summarizes current findings on the application of MSC-based therapy for colon cancer, with a focus on the mechanisms of action of MSCs as therapeutic agents, including paracrine action, the capacity of migration, and impact on the regulation of the human immune system. It also discusses strategies to improve MSCs therapeutic effectiveness through genetic engineering and potential risks that should be considered before clinical use.
Keywords
Mesenchymal stem cells; Colorectal cancer; Colon cancer; Colon cancer stem cell; Tumor microenvironment
Introduction
Colorectal cancer (CRC) is the third most common cancer worldwide, accounting for nearly two million cases in 2020 with approximately 50% mortality [1-2]. Also referred to as colorectal adenocarcinoma, CRC typically emerges from the glandular epithelial cells of the large intestine [2]. Colorectal carcinogenesis is driven by the sequential acquisition of genetic and epigenetic alterations during which normal colonic epithelium is converted into metastatic carcinoma. The progression of normal colorectal mucosa to adenoma to adenocarcinoma has been well defined, with initiating mutations in the adenomatous polyposis coli tumor suppressor gene (APC) and/or the DNA mismatch repair gene (MMR) [3]. Early-stage adenomas will progress to more advanced stages if mutations such as the K-Ras gene (KRAS) are expressed [4]. Consequently, loss of function of other apoptotic and regulatory genes may accrue, leading to more invasive adenocarcinomas. It is important to note that CRC is not a single disease but a heterogeneous group of malignancies, with different genetic characteristics of the tumors determining prognostic outcome and response to targeted therapies [4-5]. In colon cancer, the migratory phenotype of cancer cells leads to the development of distant metastases, for which there are limited treatment options [6]. The survival rate of patients with CRC is mainly dependent on the stage at which the tumor is first diagnosed. According to GLOBOCAN 2018 data, the 5-year survival rate for patients diagnosed with stage I colon cancer is 93.2%, which drops significantly to 8.1% for patients with stage IV [4,5]. Currently, the most common methods of therapy for CRC include surgery, chemotherapy, and radiotherapy. However, several studies have shown that conventional methods are ineffective in preventing drug resistance, disease recurrence, and metastasis [7]. Like other solid tumors, colonic tumor cells display great heterogeneity in their morphology, differing in expressed cell surface markers, chemoresistance, proliferation capacity, and tumor-promoting effects [8]. The ability to identify which tumor characteristics promote the metastatic potential of CRC remains limited, and the subsequent lack of personalized cell-based strategies leads to high rates of CRC-related mortality [6,7].
Colon cancer stem cells (CCSCs), with high multi-differentiation and self-renewal capacities, represent the most drug-resistant subset of cancer cells. The cancer stem cell theory points to this self-renewing subpopulation of cancer cells as the main drivers of CRC development, resistance, and metastasis. The ability of CSCs to resist traditional methods of therapy has been attributed to their heterogeneous and dynamic nature, which enables them to adapt to new environments and migrate to different tissues. Initially, the investigation of CRC carcinogenesis focused on epithelial cells alone, but the role of the tumor stroma and has become increasingly relevant due to its potential role in promoting colorectal tumorigenesis [6-8]. The colonic tumor microenvironment is composed of various cell types, including stromal, endothelial, and immune cell populations that influence the survival and growth of tumors [9]. Mesenchymal stem cells (MSCs) are multipotent stromal cells that act as essential constituents of the fibroblasts within the stroma. They have been shown to exert diverse suppressive actions against metastatic cells via paracrine signaling by soluble factors and by maintaining the integrity of the extracellular matrix (ECM) [9]. The significance of the interplay between cancer cells and TME is supported by Paget's 1989 "seed and soil" theory that a cancer cell will only proliferate when the tumor microenvironment is suitable. The microenvironment undergoes pathological changes that provide cancer stem cells with "soil" that contributes to the complexity of colon cancer. The dynamic interaction between CCSCs and the tumor microenvironment plays a critical role in regulating CCSC self-renewal [9-10]. Therefore, understanding the stromal-epithelial interactions in colon cancer is crucial to develop novel therapeutic agents that will take into account the extensive heterogeneity seen in CRC [11].
Colon Cancer Stem Cells
Colorectal cancer comprises heterogeneous cell populations that differ in gene expression and tumor-promoting activities [13]. While colon cancer stem cells (CCSCs) represent a small fraction of the cells in heterogeneous tumors, evidence suggests they play a fundamental role in tumor initiation, progression metastasis, and cancer recurrence [8]. Recent studies have shown that colon cancer stem cells are a dynamic population of cells that are continuously altered by a number of factors, including genetic and epigenetic changes, microenvironmental factors, and interactions between tumor cells [14]. This population of cells acquires a series of mutations in specific tumor suppressor genes and oncogenes that provide them different erratic abilities, including stemness, multidrug resistance, apoptosis resistance, expression of unique cell surface markers, and enhanced DNA repair capacity [8,14]. In addition, their intrinsic property of pluripotency allows them to generate tumor cells expressing different phenotypes, resulting in the growth of the primary tumor and production of new tumors [15]. Normal intestinal stem cells and cancer stem cells share their key signaling pathways, but when the microenvironment of the stem cells is altered unfavorably, normal stem cells may transform into CSCs, leading to the formation of malignant tumors [16]. More specifically, the aberrant activation of signaling pathways such as Wnt/ β-catenin, Jack/Stat, bone morphogenetic protein (BMP), TGF-β, Notch, sonic hedgehog, epidermal growth factor receptor (EGFR)/mitogen-activated protein kinase (MAPK), NF-κB, and Akt/mTOR play essential roles in the evolution and progression of CCSCs [17]. Most of these pathways are interconnected and participate in self-renewal and in epithelial to mesenchymal transition (EMT) initiation, which plays a vital role in cancer initiation and metastasis. A deep understanding of the regulatory mechanisms, specific cell markers, heterogeneity, and signaling pathways of CCSCs and their interactions with the components of the tumor microenvironment is necessary to enhance treatment strategies for colon cancer and improve patient outcomes [15,17].
Characteristics of Mesenchymal Stem Cells
Stem cells derived from different sources vary in their proliferation, differentiation, and migration capacities, which determine their efficacy in anti-cancer treatment strategies. Mesenchymal stem cells (MSCs) are non-hematopoietic multipotent stromal cells that can differentiate into a variety of cell lineages, including osteocytes, fibroblasts, adipocytes, and chondrocytes in vitro [18]. Although MSCs were originally found in the bone marrow, they can be obtained from a wide variety of tissues, including umbilical cord blood, adult muscle, peripheral blood, placenta, and adipose tissue [19]. According to the International Society of Cell Therapy (ISCT) criteria, human MSCs express the surface markers CD105, CD44, CD90, and CD73, but do not express CD45, CD34, CD19, CD14, CD11b, CD79a, or human leukocyte antigen (HLA)-DR [20]. It is important to note that MSCs isolated from different tissues display different surface antigen molecules, which play an important role in determining the functional characteristics of MSCs. Currently, the most frequently used sources of MSCs used in clinical trials are derived from the bone marrow, adipose tissue, and umbilical cord due to accessibility, ease of isolation, and MSC-based treatment efficacy [12]. The multi-directional differentiation potential observed in MSCs is among the most critical characteristics to consider when discussing therapeutic utility because different tissue sources vary in their differentiation and proliferation capacities [11-12]. Moreover, depending on the tissue of origin, MSCs display significant differences in the levels of several paracrine factors [12]. To date, most preclinical studies have been done with BM-MSCs; however, their application in the clinical setting is limited due to decreased migration capacity and complex extraction methods [18]. The harvesting of bone marrow requires an invasive procedure that yields a small number of cells, and the number, differentiation potential, and viability of bone-marrow-derived MSCs are dependent on donor characteristics, such as patient age [18]. In recent years, MSCs derived from adipose tissue have become highly attractive due to the ease of isolation, high initial cell yields, and favorable proliferation ability in vitro. Studies have revealed that the expansion and differentiation capacity, as well as the immunophenotype of adipose tissue-derived MSCs (AD-MSCs), is nearly the same as that seen in bone-marrow-derived MSCs (BM-MSCs) [12]. Umbilical cord blood-derived MSCs (UCB-MSCs) exhibit additional biological advantages relative to other adult sources, including their capacity to withstand longer culture times and enhanced anti-inflammatory effects [18]. Research has also shown that MSCs taken from birth-related tissues demonstrate increased expansion and engraftment capacity in target tissues.
MSCs have become an attractive tool within regenerative medicine due to many of their intrinsic properties, such as their ease of extraction/isolation, their ability to differentiate into almost any end-stage lineage cells, and their immunological properties, including anti-inflammatory, immunoregulatory capacities [18,20]. Also, with the ability to home into tumor sites and secrete cytokines via paracrine activity, MSCs can be recruited as biological vehicles to deliver anti-tumor drugs and therapeutic agents [11]. However, due to the differences in immunomodulatory function, migration capacity, and secreted cytokines of MSCs from different tissue sources, MSCs have been shown to have bidirectional effects on tumor growth and metastasis in animal models. Khakooet al., investigated the antitumorigenic effects of BM-MSCs in vivo conditions by injecting MSCs into mice with Kaposi's sarcoma and co-culturing the MSCs and sarcoma cells in vitro [21]. Results showed decreased levels of proliferation of sarcoma cells through inhibition of Akt-signaling. In contrast, Xu et al. examined the potential of BM-MSC-therapy for osteosarcoma by injection of MSCs into nude mice and found increased stimulation of tumor growth [22]. It is hypothesized that the discrepancies in results are related to different characteristics of derivation source of MSCs such as AD-MSC, BM-MSC, and UCB-MSC. Moreover, it could be due to tumor type and MSCs characteristics [20,22]. It should also be noted that MSCs derived from different sources have been shown to display different capacities to modulate the immune response and promote tissue repair. For example, UCB-MSCs exhibited higher production of TGF‐β (transforming growth factor‐β) and lower levels of VEGF‐α (vascular endothelial growth factor‐α) and EGF (epidermal growth factor) relative to AD-MSC, suggesting distinct pro-angiogenic and immunomodulatory potential among the different tissue-specific MSC subpopulations [11,20]. In the following sections, the primary mechanisms of therapy of MSCs for colon cancer are discussed.
MSCs Mechanisms of Therapy
The therapeutic capacity of MSCs is largely dependent on their ability to migrate, adhere, and engraft into damaged tissues [23]. Upon entry into the tumor microenvironment, the engrafted cells secrete a variety of bioactive molecules such as growth factors, cytokines, and extracellular vesicles that can exert anti-inflammatory, immunosuppressive, anti-apoptotic, and angiogenic effects [23-24]. Malignant tumors and their associated microenvironments induce MSC migration and homing via mechanisms that depend on the secretion of various inflammatory cytokines, chemokines, and growth factors [25]. There is a solid and well-established correlation between chronic inflammation and the onset of colorectal cancer, which has led many researchers to compare the tumor niche to "a wound that does not heal [26]." Thus, the factors that organize the recruitment of MSCs to the TME are likely similar to that of the injured tissue to counteract inflammation and promote healing [25-26]. Although the molecular mechanism behind the tumor homing of MSCs is still not fully understood, research has shown that various cytokines and their receptors regulate their migration capacity. Recent studies investigating the recruitment of MSCs to tumors have identified the significance of different signaling molecules in this mechanism, such as CXCR4, CXCR12, CXCCL12, MCP-1, and VCAM-1 [26]. For example, Ma et al., demonstrated that a subpopulation of MSCs expresses the C-X-C chemokine receptor type 4 (CXCR4) that binds to its ligand, CXCL12, to mediate cell migration [23]. Gaoet al., provided further support by identifying the CXCR4/SDF1 signaling axis as an important stimulus for MSC migration towards tumor-cell conditioned media [27]. In the context of colon cancer, recent studies have suggested that MSCs are recruited from the bone marrow to the stroma of developing tumors, where they serve as integral components of the tumor microenvironment [28]. Aside from their effects on the TME, MSCs have been shown to modulate CRC cell growth via other mechanisms, including angiogenesis, metastasis, and suppression of apoptosis. BM-MSCs pretreated with inflammatory cytokines IFN-α and TNF-α can elicit VEGF expression secreted by CRC cells and promote angiogenesis in vitro. Hogan et al., revealed that a higher presence of MSC-secreted PAI-1 level significantly increased the migration and proliferation of HT-29 colon cancer cells [6,33]. While many studies have produced results showing MSCs ability to promote CRC progression, the effect of MSCs remains controversial. As noted in the review of Hogan et al., the HT-29 CRC cells displayed increased proliferation, while the HCT-116 cell line showed decreased growth [6]. These results provide another example of the dynamic role that MSCs have in colonic tumors.
Nishikawa et al., demonstrated that human MSCs secreted high levels of CCR5 ligands (i.e., CCL3, CCL4, and CCL5) and that they promoted CRC growth in vivo via increased expression of CCR5. Moreover, researchers found that the CCR5 inhibitor eradicated the MSC-induced tumor growth in vivo. These results suggest that the inhibition of MSC-CRC interactions may provide the possibility of a novel therapeutic strategy for CRC [25-26]. In vitro experiments analyzing the tropism toward parental colon cancer cells CD133−/CD44− and colon cancer cells CD133+/CD44+ found that BM-MSCs migrated to CD133+/CD44+ colon cancer cells more readily, suggesting that CCSCs have an increased ability to recruit BM-MSCs into the tumor sites. After co-culture with CD133+/CD44+ colon cancer cells, BM-MSCs secreted significantly higher levels of interleukin 8 (IL-8), which is associated with liver metastases and poor treatment outcomes in patients with CRC [29]. In addition, BM-MSCs were significantly transformed into cancer-associated fibroblasts (CAF) after being recruited by CCSCs. It was observed that IL-8 secreted by BM-MSCs promoted the homing of MSCs to colon CSCs by binding to CXCR2. This idea led researchers to explore the effects of inhibiting of IL-8/CXCR2. Results showed that inhibition of IL-8 in BM-MSC reduced the migration of BM-MSC toward colon CSC populations. These findings highlight the dynamic role that MSCs can play in the progress of tumor growth and metastasis and provide an additional benefit of using BM-MSCs as cell-based carriers of anti-cancer drugs to specifically target CCSCs within the heterogeneous tumor mass [22, 29].
One of the most critical mechanisms underlying MSC-based therapy for CRC is paracrine activity, which involves the secretion of various soluble factors to exert immunomodulatory, angiogenic, and anti-apoptotic effects [25,31]. Aside from their differentiation potential, MSCs can secrete specific trophic factors such as growth factors and cytokines that modulate the activity of adjacent cells. This paracrine activity includes the secretion of soluble molecules such as growth factors, cytokines, chemokines, and the release of extracellular vesicles that contain a wide variety of molecules such as mRNA, proteins, and microRNAs. Because of their paracrine function, mesenchymal stem cells exhibit immunosuppressive properties that promote tumor targeting in heterogeneous malignancies, thereby circumventing current obstacles in gene therapy [30].
In the correlation between colon cancer pathogenesis and inflammation, it was reported that chronic inflammation plays a significant role in colorectal carcinogenesis [25]. While many studies have shown the pro-tumorigenic effects of MSCs on colon cancer, recent investigations have observed the ability of MSCs to inhibit inflammation. Following a stimulus, MSCs can secrete immunoregulatory factors or enhance the immune response. For example, He et al.,proved that IL-1β-primed MSCs could improve inflammatory disorders on dextran sulfate sodium-induced colitis [31]. Francesco and colleagues demonstrated that MSCs exhibit a potent therapeutic function in colitis-associated CRC by decreasing the expression of Ki67 through inhibition of the Smad2 signaling pathway [32]. In contrast, Wu et al.,reported that MSCs greatly enhanced CRC progression via AMPK/mTOR-mediated NF-κB activation [31]. The contradictory results in MSC clinical application may be due to the heterogeneity of MSCs, which is the main factor that restricts their application in cancer therapy. The heterogeneity of MSCs is influenced by key parameters of MSCs, including donor origin, tissue origin, passage number, expansion dose, and delivery dose [14]. Furthermore, several factors, including the culture condition, the exact disease intended to be targeted, and the local conditions of administration, may also affect the immunomodulatory function of MSCs [32].
MSCs as Vehicles for Cancer Treatment
Among the different types of MSC-based therapies, the use of MSCs are vehicles to deliver therapeutic factors represents an important step toward more efficient cancer treatments. Many of the commonly used chemotherapy drugs lack tumor specificity, and the necessary doses to reach therapeutic levels are often cytotoxic to other healthy tissues [25]. MSCs are widely used as vehicles for carrying anti-cancer agents or specific genes into tumor sites for the treatment of CRC [33]. In light of the tumor tropism properties of MSCs, they can be modified to deliver specific anti-cancer agents to tumor sites. There are many existing methods used to alter MSCs, with the most common mechanism being genetic engineering. Exosomes are vesicles released by cells that can be manipulated to encapsulate various anti-cancer agents, including proteins, miRNAs, or small drugs [32]. By using MSC-derived exosomes as delivery vehicles, researchers look to exploit their tumor homing abilities to inhibit cancer development. In addition, these vesicles are comprised of cell-free material, which decreases the risk of mutations within the cell and the risk of immune recognition by the host system [31]. MSC-derived exosomes have also been shown to play a significant role in the communication between MSCs and surrounding cell populations [34]. For example, during in vitro culture with mononuclear cells from peripheral blood, BM-MSC-derived exosomes suppressed the secretion of the pro-inflammatory factor’s TNF-a and IL-1β, while also increasing the concentration of the anti-inflammatory factor TGF- β [34-35]. Because of their ability to modulate post-transcriptional gene expression, MicroRNAs (miRs) have gained a special interest in cancer treatment. MSCs express various miRs that can be enclosed into exosomes and delivered to injured sites to exert therapeutic effects. To take advantage of this property, MSCs have been modified to carry specific miRs with anti-tumor properties [23,35]. For example, researchers engineered MSCs to produce extracellular vesicles carrying increased levels of miR-124a, which had effective anti-tumor action in glioma stem cell lines [23].
An in vivo study with BM-MSC-derived exosomes demonstrated how exosomes inhibited the proliferation and migration and increased the apoptosis of colon cancer cells through decreased expression of integrin alpha 2 [35]. The authors transfected miR-16-5p into the exosomes of BM-MSCs and observed significant suppression of CRC growth. In another in vivo study, AD-MSCs injected in mice treated with 5-fluorocytosine resulted in inhibition of tumor growth, suggesting that AD-MSCs have the ability to deliver CD genes to the injured site and exert anti-tumor effects. In order to induce anti-tumor effects, many studies have transduced MSCs with interferon-γ, interferon-β, TNF-related apoptosis-inducing ligand (TRAIL), or chemokine ligand expressing vectors [36]. Because of the unique biology of MSCs, they can be relatively easily transduced with adenoviral, oncoretroviral, and lentiviral vectors, which are integral for the expression of therapeutic genes in MSCs [37].
Many recent studies have employed MSCs transduced with therapeutic factors such as TRAIL, a transmembrane protein that can induce apoptosis in cancer cells [36-37]. Suicide gene therapy, also known as gene-directed enzyme prodrug therapy (GDEPT), relies on a vector to deliver a therapeutic transgene and prodrug to target the tumor cell [38]. The suicide gene is translated into an active enzyme within MSCs, which converts the prodrug to its cytotoxic form. This conversion induces the death of the MSCs as well as the death of nearby tumor cells through a mechanism known as the bystander effect. Kalimuthuet al., studied the effects of suicide-gene transplanted MSCs on colon cancer growth in mouse models using the herpes simplex virus type 1-thymidine kinase (HSV1-TK) and the drug ganciclovir. In this study, researchers used the “Tet-On-3G” protein to increase the MSCs expression of the HSV1-sr39TK suicide gene. The results showed increased apoptotic cell death in colonic tumors that were injected with MSCs expressing the HSV1-sr39TK suicide gene. Suicide gene therapy can negatively affect normal tissues, but it does not harm the healthy cells if the gene is delivered precisely to the targeted tumor cells. Therefore, using MSCs with the inducible Tet-On system to regulate transgene expression, the prodrug can specifically target colon cancer cells with reduced bystander cytotoxic effects [37-38]. Kucervaet al.,showed that AD-MSCs expressing the fusion yeast CD::UPRT gene combined with prodrug 5-FC yields potent cytotoxic effects on human colon adenocarcinoma HT-29 cells in vitro. These results suggest that MSCs derived from adipose tissue can act as delivery vehicles for prodrug converting genes in personalized cell-based cancer gene therapies [38]. Due to intrinsic properties, including their low immunogenicity and tumor homing abilities, using MSCs engineered for tumor therapy is a promising approach for colon cancer treatment. More specifically, using MSC-derived exosomes as cell-free delivery agents makes MSCs an attractive tool for developing novel strategies for non-cell-based therapies [34-37].
Future Directions
Mesenchymal stem cells have been widely investigated for their role in regenerative medicine due to their ability to migrate to damaged tissues, differentiate into various cell types, and modulate the host immune response. However, the therapeutic utility of MSCs for cancer is restricted by contradictory results that reveal both anti- and pro-tumor effects in preclinical studies [23]. Despite these findings, recent improvements in MSC-based cancer therapies bring new hope by providing highly effective, personalized anti-cancer treatments. MSCs and their exosomes have been extensively studied for their role in carrying therapeutic agents towards colonic tumors in preclinical murine models [39]. MSC-based gene therapy that specifically targets the tumor has improved therapeutic efficacy due to the ability of MSCs to migrate to primary and metastatic tumor sites and activation as a minipump that continuously releases various anti-tumor agents into the TME [33-36].
Because of their potential for differentiation, tumor homing capacity, and ability to produce immunomodulatory, angiogenic, and anti-apoptotic factors, MSCs have exhibited promising clinical potential for the treatment of advanced malignancies [8,11]. However, exogenous tissue-derived MSCs such as BM-MSCs, AD-MSCs, and UCB-MSCs, have all displayed a tendency to promote tumor formation and subsequent metastasis in animal models. Also, MSCs are a primary source of reactive stromal cells that increase cancer progression in the tumor microenvironment [7-10]. It is also important to note that MSCs have a limited proliferation potential and considerable donor variations. Even though MSCs can be isolated and cultured relatively easily, their life span is often dependent on host characteristics, and it can be a challenge to expand them to the high number that is required for clinical trials [23]. Moreover, efficient engraftment into tumor sites and the viability of delivered MSCs are obstacles that limit their therapeutic application. To address the limitations that hinder the potential applications of MSCs for cancer therapy, researchers have investigated the efficacy of MSCs derived from human induced pluripotent stem cells (iPSCs). Results showed that, compared with BM-MSCs, iPSC-MSCs exhibit the same tumor tropism ability but do not promote EMT, invasion, or the stemness of cancer stem cells [40]. Moreover, iPSC-MSCs have been shown to expand more rapidly and to a greater extent than BM-MSCs. In a recent clinical trial, researchers at the University of California at San Diego investigated the effects of FT500, an iPSC-derived Natural Killer (NK) cell product, in patients with advanced solid tumors. The preclinical data provides compelling evidence that this novel strategy to produce pluripotent stem-cell-derived NK cells can effectively eradicate cancerous cells in cell culture and murine models. Although the concept of using iPSC-MSCs is in its early stages, iMSCs are emerging as a new source of MSCs that could replace adult MSCs in regenerative medicine [41].
Conclusion
This review emphasizes the therapeutic role of mesenchymal stem cells and discusses their clinical applications as well as their risks in the treatment of colorectal cancer. Despite recent advances in screening, diagnosis, and treatment, there has been limited success in treating colon cancer with therapeutic agents that solely target cancer cells, especially in advanced tumors [25]. With our current understanding of colorectal cancer stem cells, it is clear that conventional methods of treatment only induce partial or incomplete remission. Surgical excision of localized disease is currently the only effective management strategy being employed against this heterogeneous population of cells [2]. Therefore, there is an urgent need to develop novel therapies that can target this unique population of CCSCs [1-5]. In order to design completely effective treatment strategies to fight colon cancer, it is imperative to understand the biology of CCSCs and their interactions with the TME. Research has shown that CCSC formation is a result of accumulated intrinsic mutations and deregulated signaling pathways within the TME. It was also regarded that colonic tumor growth and metastasis are highly dependent on the presence of CCSCs in the tumor and the components of their microenvironment. Because of this extensive heterogeneity, a complete and effective treatment must target CCSC, non-CCSCs, and elements of the TME [8]. MSCs have emerged as a promising candidate for clinical application due to their multi-differentiation potential, tumor homing capacity, and immunoregulatory properties. The ability of MSCs to migrate to the sites of tumors while escaping immune surveillance is extremely promising for personalized cell-based cancer treatments. However, the inherent qualities that make MSCs attractive tools for anti-cancer strategies have also been shown to support tumor progression and angiogenesis in CRC. Current research approaches utilize various methods to isolate and expand MSCs, and results show considerable variation among the tumor models used. The time of MSC administration, site of tumor injection, the proportion of each cell type, route of MSC administration, and length of follow-up before animal sacrifice all affect the outcome of these MSC-based interventions. At present, genetically modified MSCs have been utilized for colon cancer treatment. However, the effects of these strategies have not been proven in humans in vivo. MSCs have been widely studied and applied in many clinical trials within the field of regenerative medicine. However, the exact mechanism underlying the interaction between MSCs and the colonic tumor is still misunderstood. There is a need for further investigation into the dynamic interplay between MSCs and CCSCs, and the role of MSCs in CRC progression will allow for safer and more effective treatment methods in the future.
References
- Rawla P, Sunkara T, Barsouk A. (2019) Epidemiology of colorectal cancer: incidence, mortality, survival, and risk factors. PrzGastroenterol. 14(2):89-103.
- Biller LH and Schrag D. (2021) Diagnosis and Treatment of Metastatic Colorectal Cancer: A Review. JAMA 325(7):669-85.
- Lannagan TR, Jackstadt R, Leedham SJ, Sansom OJ. (2021) Advances in colon cancer research: in vitro and animal models. CurrOpin Genet Dev. 66:50-56.
- Montminy EM, Jang A, Conner M, Karlitz JJ. (2020) Screening for Colorectal Cancer. Med Clin N Am 104:1023-1036.
- Nguyen HT, Duong HQ. (2018) The molecular characteristics of colorectal cancer: Implications for diagnosis and therapy (Review). OncolLett. 16(1):9-18.
- Hogan NM, Joyce MR, Murphy JM, Barry FP, O’Brien T, et al. (2013) Impact of Mesenchymal Stem Cell secreted PAI-1 on colon cancer cell migration and proliferation. BiochemBiophys Res Commun. 435(4):574-579.
- Dzobo K, Senthebane DA, Ganz C, Thomford NE, Wonkam A, et al. (1896) Advances in Therapeutic Targeting of Cancer Stem Cells within the Tumor Microenvironment: An Updated Review. Cells 9(8):1896.
- Jahanafrooz Z, Mosafer J, Akbari M, Hashemzaei M, Mokhtarzadeh A, et al. (2020) Colon cancer therapy by focusing on colon cancer stem cells and their tumor microenvironment. J Cell Physiol 235:4153-166.
- Alkasalias T, Moyano-Galceran L, Arsenian-Henriksson M, Lehti K. (2018) Fibroblasts in the Tumor Microenvironment: Shield or Spear? Int J Mol Sci. 19(5):1532.
- Bahrami A, Khazaei M, Hassanian SM, ShahidSales S, Joudi-Mashhad M, et al. (2017) Targeting the tumor microenvironment as a potential therapeutic approach in colorectal cancer: Rational and progress. J Cell Physiol. 233(4):2929-36.
- Saeedi P, Halabian R, Fooladi AA. (2019) A Revealing review of mesenchymal stem cells therapy, clinical perspectives and Modification strategies. Stem Cell Investig6:34.
- Fan XL, Zhang Y, Li X, Fu QL.(2020) Mechanisms underlying the protective effects of mesenchymal stem cell-based therapy. Cell Mol Life Sci. 77(14):2771-794.
- Munro MJ, Wickremesekera SK, Peng L, Tan ST, Itinteang T. (2018) Cancer stem cells in colorectal cancer: a review. J ClinPathol. 71:110-116.
- Hirata A, Hatano Y, Niwa M, Hara A, Tomita H. (2019) Heterogeneity in Colorectal Cancer Stem Cells. Cancer Prev Res. 12(7):413-420.
- Gupta R, Bhatt LK, Johnston TP, Prabhavalkar KS. (2019) Colon cancer stem cells: Potential target for the treatment of colorectal cancer. Cancer BiolTher. 20(8):1068-1082.
- Ayob AZ, Ramasamy TS. (2018) Cancer stem cells as key drivers of tumor progression. J Biomed Sci. 25(1):20.
- Pan T, Xu J, Zhu Y. (2017) Self-renewal molecular mechanisms of colorectal cancer stem cells. Int J Mol Med. 39:9-20.
- Javan MR, Khosrojerdi A, Moazzeni SM. (2019) New Insights IntoImplementaion of Mesenchymal Stem Cells in Cancer Therapy: Prospects for Anti-angiogenesis Treatment. Front Oncol9:840.
- Hong IS, Lee HY, Kang KS. (2014) Mesenchymal stem cells and cancer: friends or enemies? Mutat Res. 768:98-106.
- Marofi F, Vahedi G, Biglari A, Esmaeilzadeh A, Athari SS. (2017) Mesenchymal Stromal/Stem Cells: A New Era in the Cell-Based Targeted Gene Therapy of Cancer. Front Immunol 8:1770.
- Iplik ES, Ertugrul B, Kozanoglu I, Baran Y, Cakmakoglu B. (2018) An answer to colon cancer treatment by mesenchymal stem cell originated from adipose tissue. Iran J Basic Med Sci. 21:465-68.
- Li JN, Li W, Cao LQ, Liu N, Zhang K. (2020) Efficacy of mesenchymal stem cells in the treatment of gastrointestinal malignancies. World J GastrointestOncol12(4):365-82.
- Hmadcha A, Martin-Montalvo A, Gauthier BR, Soria B, Capilla-Gonzales V. (2020) Therapeutic Potential of Mesenchymal Stem Cells for Cancer Therapy. Front BioengBiotechnol8:43.
- François S, Usunier B, Forgue-Lafitte ME, L'Homme B, Benderitter M, et al. (2018) Mesenchymal Stem Cell Administration Attenuates Colon Cancer Progression by Modulating the Immune Component within the Colorectal Tumor Microenvironment. Stem Cells Transl Med. 8(3):285-300.
- Sun Z, Wang S, Zhao RC. (2014) The roles of mesenchymal stem cells in tumor inflammatory microenvironment. J HematolOncol. 7:14.
- O'Malley G, Heijltjes M, Houston AM, Rani S, Ritter T, et al. (2016) Mesenchymal stromal cells (MSCs) and colorectal cancer: a troublesome twosome for the anti-tumour immune response? Oncotarget. 7(37):60752-774.
- Gao H, Priebe W, Glod J, Banerjee D. (2009) Activation of signal transducers and activators of transcription 3 and focal adhesion kinase by stromal cell-derived factor 1 is required for migration of human mesenchymal stem cells in response to tumor cell-conditioned medium. Stem Cells. 27:857-65.
- Nishikawa G, Kawada K, Nakagawa J, Toda K, Ogawa R, et al. (2019) Bone marrow-derived mesenchymal stem cells promote colorectal cancer progression via CCR5. Cell Death and Disease.10:264.
- Ma X, Chen J, Lui J. (2019) IL-8/CXCR2 mediates tropism of human bone marrow-derived mesenchymal stem cells toward CD133+/CD44+ Colon cancer stem cells. J Cell Physiol. 236:3114-128.
- Alkhuriji AF, Alsaiari SG, Alomar SY, Alnafjan AA, Alobaid H, et al. (2020) Effect of mesenchymal stem cells on cytochrome-c release and inflammation in colon cancer induced by 1,2-dimethylhydrazine in Wistar albino rats. Biosci Rep. 41(3):2020.
- Ocansey DKW, Qiu W, Wang J, Yan Y, Qian H, et al. (2020) The Achievements and Challenges of Mesenchymal Stem Cell-Based Therapy in Inflammatory Bowel Disease and Its Associated Colorectal Cancer. Stem Cells Int 18:7819824.
- Zhou Y, Yamamoto Y, Xiao Z, Ochiya T. (2019) The Immunomodulatory Functions of Mesenchymal Stromal/Stem Cells Mediated via Paracrine Activity. J Clin Med. 8(7):1025.
- Wang S, Miao Z, Yang Q, Wang Y, Zhang J. (2018) The Dynamic Roles of Mesenchymal Stem Cells in Colon Cancer. Can J GastroenterolHepatol. 2018:7628763.
- Jimenez-Puerta GJ, Marchal JA, López-Ruiz E, Gálvez-Martín P. (2020) Role of Mesenchymal Stromal Cells as Therapeutic Agents: Potential Mechanisms of Action and Implications in Their Clinical Use. J Clin Med. 9(2):445.
- Xu Y, Shen L, Li F, Yang J, Wan X, et al. (2019) microRNA-16-5p-containing exosomes derived from bone marrow-derived mesenchymal stem cells inhibit proliferation, migration, and invasion, while promoting apoptosis of colorectal cancer cells by downregulating ITGA2. J Cell Physiol 234:21380-21394.
- Melzer C, von der Ohe J, Hass R. (2018) Concise Review: Crosstalk of MesenchymalStroma/Stem-Like Cells with Cancer Cells Provides Therapeutic Potential. Stem Cells.36(7):951-68.
- Kucerova L, Altanerova V, Matuskova M, Tyciakova S, Altaner C. (2007) Adipose tissue-derived human mesenchymal stem cells mediated prodrug cancer gene therapy. Cancer Res. 67(13):6304-13.
- Kalimuthu S, Zhu L, Oh JM. Regulated Mesenchymal Stem Cells Mediated Colon Cancer Therapy Assessed by Reporter Gene Based Optical Imaging. Int J Mol Sci. 19(4):1002.
- Chu DT, Nguyen TT, Tien NLB. (2020) Recent Progress of Stem Cell Therapy in Cancer Treatment: Molecular Mechanisms and Potential Applications. Cells. 9(3):563.
- Zhao Q, Gregory CA, Lee RH, Reger RL, Qin L, et al. (2015) MSCs derived from iPSCs with a modified protocol are tumor-tropic but have much less potential to promote tumors than bone marrow MSCs. PNAS. 112(2):530-535.
- Sabapathy V, Kumar S. (2016) hiPSC‐derived iMSCs: NextGen MSCs as an advanced therapeutically active cell resource for regenerative medicine. J Cell Mol Med. 20:1571-1588.