The COVID-19 Pathway: A Proposed Oral-Vascular-Pulmonary Route of SARS-CoV-2 Infection and the Importance of Oral Healthcare Measures
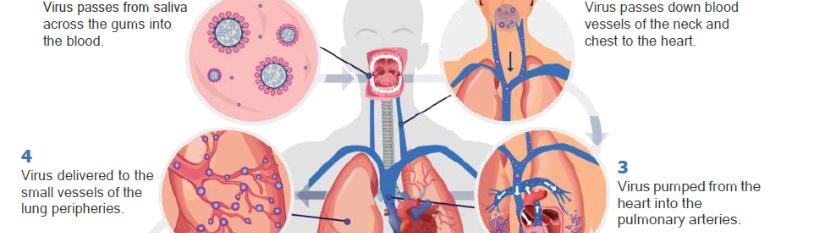
Graham Lloyd-Jones1*, Shervin Molayem2, Carla Cruvinel Pontes3 and Iain Chapple4
1Consultant Radiologist, Salisbury District Hospital, United Kingdom, Director of Radiology Masterclass
2Periodontist, Director, Mouth-Body Research Institute, Los Angeles, California
3Periodontist, Researcher, Mouth-Body Research Institute, Cape Town, South Africa
4Professor, Periodontal Research Group, Institute of Clinical Sciences, College of Medical & Dental Sciences, The University of Birmingham & Birmingham Community Health Trust, Birmingham, United Kingdom
*Corresponding author: Graham Lloyd-Jones, Consultant Radiologist, Salisbury District Hospital, United Kingdom, Director of Radiology Masterclass.
Citation: Lloyd-Jones G, Molayem S, Pontes CC, Chapple I. (2021) The COVID-19 Pathway: A Proposed Oral-Vascular-Pulmonary Route of SARS-CoV-2 Infection and the Importance of Oral Healthcare Measures. J Oral Med and Dent Res. 2(1):1-25.
Received: April 09, 2021 | Published: April 20, 2021
Copyright© 2021 genesis pub by Lloyd-Jones G, et al. CC BY-NC-ND 4.0 DEED. This is an open-access article distributed under the terms of the Creative Commons Attribution-Non Commercial-No Derivatives 4.0 International License., This allows others distribute, remix, tweak, and build upon the work, even commercially, as long as they credit the authors for the original creation.
Abstract
Since the emergence of severe acute respiratory syndrome coronavirus 2 (SARS-CoV-2) infections in late 2019, the world has faced a major healthcare challenge. There remains limited understanding of the reasons for clinical variability of coronavirus disease 2019 (COVID-19), and a lack of biomarkers to identify individuals at risk of developing severe lung disease. This article aims to present a hypothesis on a vascular route of transfer of SARS-CoV-2 from the oral cavity to the lungs. Saliva is a reservoir of SARS-CoV-2, thus any breach in the immune defenses of the mouth may facilitate entrance of the virus to the vasculature through the gingival sulcus or periodontal pocket. From the oral vasculature, the virus would pass through veins of the neck and chest, and reach the heart, being pumped into pulmonary arteries, and to the small vessels in the lung periphery. The binding of the virus to the angiotensin-converting enzyme 2 receptor (ACE2), present on the endothelial surface of lung vessels, inactivates ACE2 and increases angiotensin-II levels, leading to pulmonary vasoconstriction and immunothrombosis (inflammatory-mediated clotting). This leads to vascular congestion, proximal vasodilatation, and subsequent lung parenchymal damage mediated by endothelial dysfunction. The biological rationale for the oral-vasculo-pulmonary route of infection is discussed in detail in this article, including pertinent radiological and oral cavity scientific findings. We propose that dental plaque accumulation and periodontal inflammation would further intensify this pathway. Therefore, it is suggested that daily oral hygiene and oral healthcare should be prioritized as such measures could be potentially lifesaving for COVID-19 patients. If this proposed pathological pathway is verified, it would be hugely significant in terms of understanding disease management. Simple low-cost measures, such as use of specific mouthwashes, could decrease the salivary viral load, and help prevent or mitigate the development of lung disease and severe COVID-19.
Keywords
Severe acute respiratory syndrome coronavirus 2 (SARS-CoV-2); Saliva; Periodontal inflammation; Oral healthcare; Mouthwash; Lung disease; Disease management; COVID-19 pneumonia
Introduction
Since the first cases of SARS-CoV-2 infection emerged in China, the world has faced a major ongoing healthcare challenge. Although there have been advances in treatments and vaccination in populations, there remains limited understanding of the reasons for clinical variability of the disease. There is a lack of effective biomarkers to identify individuals at risk of developing COVID-19 lung disease, or those who might progress to severe disease leading to intensive care admission, mechanical ventilation, or death [1]. In this article, we propose a novel understanding of SARS-CoV-2 transmission from the mouth to the lungs and the development of COVID-19 lung disease (Figure 1).
Our hypothesis is based upon:
- Radiological evidence for primary vascular pathological processes in the lungs;
- An understanding of the upper respiratory tract as the initial site of infection;
- The formation of a viral reservoir in the oral cavity (and saliva);
- Potential for translocation of the virus from saliva to the gingival sulcus/periodontal pocket;
- Survival of the virus within the sub-gingival plaque biofilm, thus evading the oral mucosal immune response;
- Subsequent direct vascular delivery to the pulmonary vessels;
- A model of the biological processes associated with viral binding of the ACE2 receptor on the endothelium of pulmonary vessels and how subsequent processes correlate with the radiological features of a primary pulmonary vasculopathy.
If confirmed, this hypothetical model may provide a rationale for understanding why some individuals develop COVID-19 lung disease and others do not. It would also fundamentally change the way COVID-19 is managed, providing a new line of exploration into treatments targeted at the source of the viral reservoir, the mouth.
Figure 1: The COVID-19 pathway: A hypothetical model for the oral-vascular-pulmonary route of infection.
Initial Upper Respiratory Tract Infection and Proposed Mechanism of Transmission to the Lungs via Blood Vessels
Based on knowledge of the intensity of expression of the main SARS-CoV-2 binding receptor – the ACE2 receptor – the upper airways are considered the initial site of infection for SARS-CoV-2, rather than the lower respiratory tract. Expression of the ACE2 receptor is reported to be between 200 to 700 times more intense in the nasal airways, specifically on the surface of the olfactory neuroepithelial cells, compared to the respiratory epithelial cells of the lower respiratory tract [1,2]. The conclusion from the study by Chen and Shen et al. [2] that the initial site of infection is the upper airway, challenges the notion that the SARS-CoV-2 virus is necessarily delivered to the lungs via the airways, where expression of ACE2 receptors on respiratory epithelial cells is low [3].
The concept that the upper airways may be the predominant initial site of infection for viral transmission to the lungs requires further scrutiny. Here we present radiological evidence that raises the important possibility of a vascular viral delivery route to the lungs rather than via the respiratory airways. The proposed model describes the oral cavity as the reservoir of SARS-CoV-2, specifically in saliva, with transmission to the lungs mediated by a breach of the mucosal immune defense barrier of the periodontal tissues or oral mucosa, with subsequent intravascular carriage. If proven to be correct, this hypothesis would have significant implications for the understanding of how the disease should be managed. Simple antimicrobial oral healthcare measures could be implemented not only with the aim of reducing the risk of transmission between individuals but also with the aim of providing benefit to individuals who are COVID-19 positive. Specifically, these measures could be a means of mitigating the risk of developing lung disease, and therefore the most severe form of the disease.
Radiological Perspective – COVID-19 Lung Disease
1. Pathological Distribution of Disease
Pulmonary radiological findings in COVID-19 do not align with a model of SARS-CoV-2infection primarily causing disease of the airways of the lungs; the initial and dominant pathological features demonstrated radiologically are vascular in nature [4-6].
The distribution of lung disease does not favor an inhaled pathogen. No known inhaled infective pathogen has preferential tropism for the periphery of the lung bases. Rather, inhaled pathogens would be expected to present a uniform distribution to other areas of the lungs, including the mid or upper areas, and would not be expected to spare the perihilar or central areas [7,8].
It has also been noted that many of the radiological findings typically associated with respiratory pneumonia, for example bronchial wall thickening, mucous secretion, and the ‘respiratory tree-in-bud’ opacification of small airways, are not features of COVID-19 [4,9]. Furthermore, if the airway findings typically associated with respiratory pneumonia are present on computed tomography (CT), they are considered inconsistent with the diagnosis of COVID-19 [10].
2. Evidence of Pulmonary Vascular Phenomena
Conversely, there are numerous studies within the radiological literature describing the pathogenesis of COVID-19 lung disease as driven by vascular phenomena [4-6,9-14]. Early in the pandemic period, the presence of “ground-glass opacities” visible on CT was reported to be the hallmark sign of COVID-19 lung disease [15]. However, these ground-glass opacities were acknowledged as a non-specific feature, and histological confirmation of their significance was required, with edema or hemorrhage suggested as possible causes [16]. Notably, the radiological literature now reports that these ground-glass opacities are accompanied by abnormally dilated blood vessels (), which are thought to be responsible for the phenomenon of pulmonary arteriovenous vascular shunting and subsequent hypoxemia [4].
Figure 2: CT images of patients with COVID-19 lung disease demonstrating ground-glass opacities (yellow arrows) accompanied by abnormally dilated blood vessels (green arrows).
A specific vascular feature known as the ‘vascular tree-in-bud’ sign (not to be confused with ‘respiratory tree-in-bud’ found in conventional respiratory pneumonia) is visible on CT as a distinct entity in 64% of patients with COVID-19 lung disease [11]. This sign is thought to be a marker of the pathological process of immunothrombosis and can be visible without lung parenchymal changes in the form of ground-glass opacities. The presence of this sign correlates with the length of hospital stay [12].
Further evidence of vascular disease comes from studies of Dual-Energy CT which describe perfusion defects in 100% of patients with COVID-19. These defects of blood flow are categorized by two distinct patterns: a wedge-shaped pattern – analogous to pulmonary embolism; and a mottled/amorphous pattern – analogous to chronic or idiopathic thromboembolic hypertension. Dilated blood vessels and hyperperfusion are also described proximal to areas of ground-glass opacification [13].
3. Distinct Phenotype of Thromboembolic Disease
There has been much interest regarding the high incidence of pulmonary thromboembolic disease in COVID-19 patients. When compared to conventional pulmonary thromboembolic disease, a different distribution is described in patients with COVID-19. In COVID-19, the filling defects visible within pulmonary arteries with CT pulmonary angiography (CTPA) are lower in volume and more peripheral. This difference is thought to be related to the pathological process of immunothrombosis [14]. Indeed, immunothrombosis is the main driver of disease in the lungs [15-17], and can even be considered as an appropriate immune response, serving to trap pathogens in the affected area of tissue, thus preventing escape into the systemic circulation [18]. This difference in the distribution of thromboembolic disease, with smaller and more peripheral filling defects visible on CTPA, is significant because it is known that smaller and more peripheral clots are more likely to result in pulmonary vascular occlusion when compared to larger central filling defects [19]. Many peripherally-located areas of ground-glass opacification are morphologically identical to pulmonary wedge-shaped infarcts (). These are visible regardless of the presence or absence of visible filling defects in adjacent pulmonary arteries [20].
Figure 3: CTPA scans of the lungs in patients with COVID-19 lung disease. Wedge-shaped areas of ground-glass opacification located at the edges of the lungs resemble pulmonary infarcts. These can be present with or without visible filling defects in the pulmonary arteries on CTPA examination.
4. Correlation of Radiological and Autopsy Findings
It is also important to note that both macroscopic and microscopic pulmonary vascular obstruction is found on autopsy and that pulmonary infarcts are indeed present in the majority of individuals dying with COVID-19 lung disease [21]. Viral elements have been detected in endothelial cells in autopsy studies of those who have died with COVID-19, with evidence of endothelial cell inflammation and inflammatory cell death [22,23]. Histologically, microangiopathy of lung vessels is described with microthrombi visible within both pulmonary arterioles and peripheral lung venules. Thus, there is thrombosis on both sides of the capillary bed of the pulmonary vasculature, proximal and distal to the alveolar capillaries [24]. It is important to appreciate that this means that CTPA, the conventional imaging modality used to look for pulmonary thromboembolic disease, will underestimate the presence of thrombosis because some of the thrombosis is on the venous side which is not enhanced with intravenous contrast. This may be helpful in understanding the pathogenesis of some of the peripheral vasculitis mimics seen COVID-19 lung disease which thought to be mediated by microemboli arising from the venous side of the pulmonary vasculature (blood vessels returning to the heart).
In summary, the radiological findings are not consistent with dominant or primary airways disease but rather are entirely consistent with a disease of the lung blood vessels occurring first. This vascular disease is mediated by the process of immunothrombosis which below is proposed to result from interaction with the pulmonary endothelial ACE2 receptor. The consequences of this interaction and subsequent effects of deregulated increase of angiotensin-II and vascular congestion could explain the other radiological features of proximal vascular dilatation and the vascular tree-in-bud sign. The development of ground-glass opacities could result from endothelial dysfunction [4]. Indeed, endothelial dysfunction is widely reported as a dominant pathological feature [26,27].
Periodontal Perspective – COVID-19 in the Oral Cavity
1. Entry factors for SARS-CoV-2 in oral and gingival tissues
The invasion of host cells by SARS-CoV-2 is mediated by ACE2 receptors, furin, and trans membrane protease serine 2 (TMPRSS2). Viral spike proteins bind to ACE2 receptors on the surface of host cells, and TMPRSS2 mediates endocytosis. Furin is involved in the release of new viral particles to the extracellular compartment [28]. These mediators, which are key elements for infection, are expressed abundantly in the nasal airways and oral cavity, including gingival tissues, minor salivary glands, and tongue [29,30]. Although not all oral tissues express the three mediators of viral entry, cells of the sulcular epithelium do express ACE2, TMPRSS2, and furin. This indicates the potential for the gingival sulcus to be a target for SARS-CoV-2 infection. Thus, several niches in the oral cavity can become infected by the virus, including the gingival sulcus [30,31].
2. Presence of SARS-CoV-2 in the oral cavity and periodontal tissues
There is strong evidence from several studies confirming the presence of SARS-CoV-2 in saliva, minor salivary glands, tongue, and gingival crevicular fluid [32]. A recent study on autopsy tissues from deceased COVID-19 patients reported viral infection of the oral mucosa and salivary glands [29]. Another post-mortem study confirmed the presence of viral RNA in the periodontal tissues of 5 out of 7 patients who died of COVID-19 [33]. Together, these findings suggest that SARS-CoV-2 is abundant in saliva and may infect salivary gland tissue, gingival and oral mucosal cells.
A study from Huang et al. [34] suggests that the virus can persist in saliva or in the nasopharynx for over two months. In asymptomatic individuals, viral clearance was observed after 0.5 to 3.5 weeks [34]. The salivary viral load has been linked to loss of taste, overall disease severity and mortality, being a better predictor of poor outcome than patient age or viral load in the nasopharynx [34,35]. This is particularly important given that patient age is considered the most significant risk factor. [36]. Although it could be speculated that the viral load in saliva is merely a marker for generalized high viral load, this does not explain why the viral load in the nasopharynx is not a predictor of poor outcome. In the study from Huang et al. [34] most asymptomatic individuals displayed only nasopharyngeal swab positivity. Saliva viral load seems to be a specific predictor of poor outcome [35].
3. Periodontal pockets as a reservoir for viruses
Previous studies have reported the presence of human viruses in saliva, gingival crevicular fluid (GCF), subgingival plaque, and gingival tissues, including human immunodeficiency virus, herpesviruses, Epstein-Barr virus, cytomegalovirus, and Zika virus [37-41]. In a recent SARS-CoV-2 study, viral RNA was detected in the GCF of 64% of COVID-19 positive patients [32]. It has been speculated that viral particles in the oral cavity can migrate into the gingival sulcus/periodontal pockets, where the conditions are favorable for their survival. Sub-gingival plaque biofilm can provide a unique environment for viruses, and in periodontitis patients, the periodontal pocket epithelium develops micro-ulcerations that facilitate the passage of microorganisms and viral particles to the underlying connective tissue and gingival capillary complex, reaching the systemic circulation [31,33]. The area of exposed connective tissue and associated blood vessels that are in direct contact with the sub-gingival biofilm ranges from 5 cm2 in mild disease to more than 20 cm2 in severe periodontitis [42]. Indeed, peripheral blood neutrophils in periodontitis patients have been shown to exhibit a type-1 interferon gene expression signature, consistent with intravascular exposure to periodontal microorganisms such as viruses [43]. Thus, periodontal pockets possibly present suitable conditions for viral replication, infection, and spread to gingival capillaries.
Studies have shown that poor oral hygiene and periodontitis increase the risk for development of severe COVID-19 with poor outcomes [31,33,34,45]. Specifically, the recent study by Marouf et al. [46] examined dental X-rays of a large population sample (568 patients) with COVID-19. The study found an increased risk of developing severe COVID-19 (defined as intensive care admission, need for mechanical ventilation, or death) in those with periodontitis, with an overall odds ratio of 3.67 after confounders were accounted for, including age, sex, smoking, BMI, diabetes and comorbidities [46].
4. Oral and nasal cavities as entry points for microorganisms
In periodontitis patients, the risk for viral invasion is likely to increase due to potential disruption of the pocket epithelium resulting from local inflammation, which is the same principle that potentially explains bacterial entrance to the systemic circulation [47]. Even in healthy patients, the permeable nature of the junctional epithelium can facilitate viremia [48].
The presence of oral bacteria in the systemic circulation has been reported previously in studies on bacteriemia and infective endocarditis of oral origin. These studies propose that oral bacteria can cause damage elsewhere in the body, with the risk being higher with poor oral hygiene and periodontal inflammation [49]. As bacteria can pass into the systemic circulation via breakdown of the immune defenses of the mouth, then the same route could be open to viruses, including SARS-CoV-2, and facilitated by periodontal disease. Other potential sources of transmucosal transfer include the floor of the mouth (including salivary ducts) and Kiesselbach's plexus (Little’s area) of the nose, however research evidence is currently lacking and nasal symptoms such as anosmia are associated with a lower severity of coronavirus disease [50]. We propose that transfer across the gingival crevice is more likely to be the most significant pathway, rather than a secondary route.
5. The potential role for viral-bacterial synergy in the periodontal environment
A higher prevalence of cytomegalovirus, Epstein-Barr virus, and other herpesviruses has been reported in the subgingival plaque biofilm from periodontitis patients when compared to patients with gingivitis or a healthy periodontium [51,52]. The presence of herpesviruses in periodontal pockets seems to increase the risk for tissue destruction, suggesting a synergistic action between viruses and periodontal pathogens [53]. Several Gram-negative anaerobic bacteria implicated in periodontal disease have been associated with Epstein-Barr virus and cytomegalovirus, particularly Porphyromonas gingivalis and Tannerella forsythia [52]. Thus, the co-presence of SARS-CoV-2 with periodontal bacteria may exacerbate periodontal tissue damage, but the nature, extent, and consequences of this interaction are currently unknown. In periodontitis patients, it can be speculated that i) a viral-bacterial synergy might facilitate penetration of SARS-CoV-2 through the pocket epithelium, ii) such an interaction can help viruses evade the immune response, thus enabling its entrance to gingival capillaries and endovascular transmission directly to the pulmonary vessels. Co-infection in COVID-19 is also a possibility, given that serious respiratory conditions are often associated with viral bacterial co-infections [54]. However, there is a scarcity of data on SARS-CoV-2 bacterial co-infection [24]. Significantly, autopsy studies show a surprising lack of bacterial super-infection in those who have died from COVID-19 [24]. Also, a report relating to critical care patients did not find evidence of bacterial co-infection in blood, sputum, or bronchoscopic sampling upon admission to intensive care [55].
It is also possible that periodontal inflammation can increase the risk for viral infection. A study on Epstein-Barr virus found that gingival epithelial cells were frequently infected, and the level of viral infection correlated with the level of periodontal inflammation [56]. Previous studies report that P. gingivalis can facilitate the reactivation of latent Epstein-Barr and HIV-1 viruses [57,58]. Hence, a synergistic relationship between SARS-CoV-2 and periodontal bacteria cannot be excluded.
In immunocompromised mice, cytomegalovirus and P. gingivalis co-infection resulted in the highest mortality when compared to inoculation with the virus alone, P. gingivalis alone, or the combination of the virus and Escherichia coli. Based on the observed lower systemic levels of gamma interferon and lymphoid depletion observed in P. gingivalis and cytomegalovirus infection, it was suggested that this periodontal bacteria can increase the viral impact on the host [59].
6. Potential role of local and systemic inflammatory response
In periodontitis, the host response to microorganisms in the subgingival biofilm is mediated by the expression of pro-inflammatory cytokines, particularly tumor necrosis factor α (TNF-α), interleukin-1β (IL-1β), and interleukin-6 (IL-6). These soluble proteins can change cellular functions to promote and perpetuate inflammation and tissue destruction in the periodontal tissues and elsewhere in the body [60,61]. The link between periodontitis and systemic diseases has been researched extensively in the last decade, and findings from multiple studies point to the significance of elevated levels of pro-inflammatory cytokines and acute-phase proteins [62-65]. Indeed, studies have demonstrated that peripheral blood neutrophils of periodontitis patients are hyper-reactive with respect to cytokine release (IL-1β, IL-8, IL-6, TNF-α) when FcγR and Toll-like R4 receptors are challenged, relative to non-periodontitis controls [66]. Periodontal treatment has been shown to positively affect systemic inflammation in healthy patients and in those who have chronic diseases, such as type-2 diabetes [67], hypertension [68], coronary heart disease, and atherosclerosis [69-74].
Severity of COVID-19 has also been linked to systemic inflammation [75,76]. In COVID-19 patients, the risk for respiratory failure was 22 times higher in patients who presented high IL-6 levels upon hospital admission [77].
Although occult sources of infection in the body that perpetuate inflammation, such as periodontitis, contribute to the systemic inflammatory burden, there is limited evidence of overspill of locally produced inflammatory mediators in the periodontium into the systemic circulation, and no evidence for such disseminating inflammation triggering the lung disease. A direct role for the periodontal inflammatory response seems unlikely, especially in view of the potential for direct endothelial viral-ACE2 interaction as described below.
7. Links between periodontitis and oral hygiene with other respiratory conditions
Evidence suggests that periodontitis can increase the risk for respiratory diseases such as pneumonia, and chronic obstructive pulmonary disease (COPD) [78-80]. Studies also report on decreased lung volume, airflow limitation, and worse pulmonary function in systemically healthy patients with periodontitis [81,82], and patients with both periodontitis and COPD [83], as well as successful periodontal treatment resulting in reduced exacerbations of COPD [84]. COPD has been suggested to be low in prevalence in COVID-19 cases but conversely, if present, is described as a risk factor for poor outcome in COVID-19 [85,86].
In hospital settings, adequate plaque control measures and dental treatment have been shown to reduce the incidence and severity of pulmonary infection [87,88]. Aspiration and hematogenous spread of oral microorganisms have been described as potential pathways for the connection between oral and pulmonary conditions [89]. But findings from a study of the CT chest features in COVID-19 showed that the presence of airway secretions is not typical in these patients [10], which further supports the notion of a potential hematogenous route of transmission from the oral cavity to the lungs. It is also important to consider the lack of bacterial super-infection found histologically, as reported above [24,55].
There is evidence that oral hygiene measures lower the incidence of aspiration pneumonia in elderly patients in hospital and nursing homes, decreasing morbidity and mortality [90]. In the systematic review from Sjogren et al. [91] the authors estimate that one in ten cases of death from pneumonia in nursing home patients can be prevented through simple oral hygiene measures [91]. Given that periodontitis and inadequate oral hygiene negatively impacts respiratory conditions and lung function, particularly in hospitalized patients, their potential to worsen lung complications in hospitalized COVID-19 patients should not be ignored. This also perhaps acts as an existing rationale for treating any patient with symptomatic COVID-19 by implementing oral hygiene measures.
8. Shared risk factors between COVID-19 and periodontitis
Periodontitis and poor outcome in COVID-19 share many risk factors, such as patient age [92,93], male sex [94,95], diabetes [96,97], cardiovascular disease [98,99], obesity [93,100], COPD [101,102], Down syndrome [103,104], specific ethnic groups [105,106], type A blood group [107,108], chronic kidney disease [109-111], physical disability or learning difficulty [112,113] and dementia [114,115].
Smoking is a recognized risk factor for periodontitis [116]. In a recent meta-analysis, smoking was associated with increased risk for severe COVID-19 [117] but not all studies confirm this association [118]. It is notable that if COVID-19 lung disease was mediated by airways pathology, smoking would be considered a risk factor for poor outcome, as it is in influenza [119]. Rather, there is counterintuitive evidence about the role of smoking and the suggestion that nicotine may have a therapeutic role [120-122]. The harmful effects of smoking on gingival tissues are mediated, in part, by the vasoconstrictive biological action of nicotine [123], which leads to significantly reduced gingival bleeding and decreased diameter of gingival capillaries [124,125], Conceivably, the local vasoconstriction action of nicotine may limit the transfer of microorganisms across the mucosal membrane of the oral cavity and periodontal tissues to the venous drainage of the mouth.
A large population study showed increased COVID-19 symptoms in individuals who smoke [126]. Therefore, smoking may be considered yet another risk factor shared between COVID-19 and periodontitis, however the exact mechanism of interaction of smoke inhalation and nicotine action is complex and likely involves multiple toxins present within cigarette smoke vapor and tar fractions.
9. Higher severity of COVID-19 in patients with poor oral hygiene/periodontal disease
As mentioned previously, the case-control study on 568 COVID-19 patients showed an association between periodontitis and COVID-19 severity [46]. This study found that periodontitis was associated with complications for COVID-19 including death (odds ratio 8.81), intensive care admission (odds ratio 3.54), and need for assisted ventilation (odds ratio 4.57) [46]. Dental plaque could provide a constant source of viral delivery to the vasculature during the acute phase of COVID-19. Thus, it is biologically plausible that the ongoing delivery of the virus itself to the lung vessels could account for poorer outcomes, rather than viral transmission via the airways or bacterial super-infection.
Biological Rationale for the Oral-Vascular-Pulmonary Route of Infection
Here we propose a model of direct viral delivery from the oral cavity via the venous drainage of the mouth, neck (jugular veins), and chest (superior vena cava), through the right side of the heart, and then to the pulmonary vessels. Such a route of transmission would be compounded by poor oral hygiene or periodontal disease. This model of disease could be hugely significant in terms of a novel understanding of the disease pathogenesis and its management.
Below is presented the biological plausibility for this oral-vascular-pulmonary route of SARS-CoV-2 transmission from the oral cavity to the lungs facilitating the development of COVID-19 lung disease. It is proposed that the oral-vascular-pulmonary anatomical route of transmission could explain why some patients develop lung disease, and so are susceptible to severe disease, and others do not.
Anatomical Route – from the Oral Cavity to the Lungs
With high levels of SARS-CoV-2 in saliva, any breakdown of the primary immune barrier in the oral cavity could facilitate viral entry to capillaries. Poor oral hygiene could further increase the risk of infection by changing the physiologically permeable junctional and sulcular epithelium to a pocket lining epithelium, which then ulcerates creating a co-called “periodontal wound”. The size of the periodontal wound, and therefore the associated vascular access to plaque microorganisms, can be calculated and increases as oral hygiene worsens. Through the venous drainage of the mouth and neck, the virus would reach the superior vena cava, entering the right side of the heart, and then be pumped into the pulmonary arteries. This route of hematogenous delivery of SARS-CoV-2 to the lungs, rather than via the lower airways, would explain the vascular distribution of the lung disease in COVID-19 seen radiologically.
If other microorganisms can enter the systemic circulation via a breach of the mucosal defense barrier of the mouth, it should be asked why they do not evidently cause lung disease. The specific pulmonary vascular tropism of SARS-CoV-2 requires an explanation.
ACE2 Receptor-Virus Interaction
SARS-CoV-2 is known to bind to ACE2 receptors. These receptors are said to be expressed in respiratory epithelial cells of the airways which has been used to explain the conventional model of viral interaction with the lungs [127]. Although this is one way the virus could enter, the expression of ACE2 on respiratory epithelial cells has been shown to be at much lower levels when compared to nasal neuroepithelial cells [2,3], and some have suggested very low levels or even no expression in the normal respiratory system [128].
Importantly, expression of ACE2 receptors has also been reported in endothelial cells of pulmonary vessels and several other organs of the body [26,129,130]. This provides a specific model for the interaction of SARS-CoV-2 with the endothelium of pulmonary vessels, unlike other viruses, which are not known to bind to the ACE2 receptor. In view of the lack of airways disease visible radiologically, it also helps explain the question of why the pulmonary vessels are dominantly affected compared to other organs [131]. According to the model of the oral-vascular-pulmonary anatomical route of transmission from the mouth to the lungs, the virus would be delivered to the pulmonary vessels first, before it could reach other organs.
Additionally, as presented above, the radiologically observed dilated vessels and the specific vascular tree-in-bud sign, which can be visible independent of ground-glass opacification or consolidation, are consistent with a model of viral-ACE2 interaction and development of immunothrombosis and its consequences.
Simply put, if the virus could pass from saliva or the sub-gingival periodontal environment into the venous drainage of the mouth, it would first be delivered directly to the small vessels of the lung periphery. This is exactly where immunothrombosis occurs, being accepted as the main driver of the pathology [12,14,17,132].
On interacting with ACE2 receptors on pulmonary vascular endothelial surfaces, viral binding would lead to an unregulated increase in local levels of angiotensin-II hormone, which has multiple biological functions, including vasoconstriction, promotion of inflammation, and thrombosis [133]. These direct actions of angiotensin-II have been proposed as a contributor to the development of immunothrombosis in COVID-19 lung disease [134]. In this way, unregulated levels of angiotensin-II could be the trigger for immunothrombosis and act as a key pathological step in the development of lung disease and the systemic hypercoagulable state.
In COVID-19 autopsy studies, viral particles were detected in endothelial cells of the lungs, with evidence of endothelial cell inflammation and inflammatory cell death [22,23,26]. The process of immunothrombosis could be considered locally harmful because it prevents blood flow and, thus, prevents gas exchange. However, it is suggested that the process of immunothrombosis serves to contain and eliminate pathogens [18], which perhaps helps to explain the lack of viremia in the early stages of COVID-19 [135]. It can be postulated that the viremia seen later in the disease process, which is a predictor of death [136].
Clinical Significance
From the oral cavity, if SARS-CoV-2 can reach the lungs through the blood, causing immunothrombosis-driven disease in the pulmonary vessels, then early measures to decrease transmission to the lungs in this way must be considered in the management of COVID-19. This concept could influence the development of new approaches with the aim of preventing or mitigating lung disease.
This concept potentially highlights the importance of active oral healthcare and adequate daily oral hygiene measures in the management of COVID-19 [44]. Importantly, it is noted that readily available mouthwashes containing cetylpyridinium chloride (CPC) or ethyl lauroyl arginate (ELA) can inactivate SARS-CoV-2 with high efficacy in vitro [137-140]. Those containing povidone-iodine (PVP-I) have also been shown to be effective [139,141-47]. Mouthwash products containing chlorhexidine or ethanol alone displayed little or no ability to inactivate SARS-CoV-2 [138]. Given that mouthwash products containing these specific ingredients are readily available across the world, they could provide a cheap and effective treatment in those with COVID-19 with the additional potential benefit of reducing the risk of transmission to others. These products should only be used as directed by the manufacturer, or as advised by oral healthcare professionals as part of a population study or clinical trial. These specific mouthwash ingredients may potentially play a role in mitigating development or worsening of the lung disease at any stage, from those who are swab positive and asymptomatic in the community to those who are hospitalized or even in intensive care.
Media outlets have reported the adoption of mouthwashes in some countries, as advised by government officials, either officially or perhaps unofficially. In Japan, for example, the sales of mouthwash increased substantially after a governor advised the population to use a gargling solution [148]. COVID-19 outcomes in Japan are significantly better than in other G20 countries, such as the UK and the US (as of February 19th, 2021 – data from the previous seven day period – 3.41 deaths per million (Japan), 33.28 deaths per million (USA), and 46.28 deaths per million in the (UK) [149]. Although there are likely to be many confounding reasons for the lower mortality in Japan, this difference of approach is raised as a point of interest to be urgently researched by governmental and public health officials.
Recommendations for Good General Oral Healthcare
Adequate daily home oral care habits are essential for oral and general health, as it decreases the risk for dental caries, gingivitis, and periodontitis. If proven to be correct, the concept of the oral-vascular-pulmonary infection route may mean that these simple measures could reduce the risk of developing severe COVID-19 lung disease.
Although each patient has unique needs, the European Federation of Periodontology (EFP) provides general recommendations [150]:
- There is a universal recommendation to brush twice daily for at least 2 minutes with a fluoridated toothpaste.
- For periodontitis patients 2 minutes is likely to be insufficient.
- Manual or power toothbrushing is recommended as a primary means of reducing plaque and gingivitis. The benefits of toothbrushing outweigh any potential risks.
- Daily inter-dental cleaning is strongly recommended to reduce plaque and gingival inflammation. When gingival inflammation is present, inter-dental cleaning, preferably with interdental brushes should be professionally taught to patients.
- For the treatment of gingivitis and where improvements in plaque control are required, adjunctive use of antiplaque chemical agents may be considered. In this scenario, mouthwashes may offer greater efficacy but require an additional action to the mechanical oral hygiene regime.
Mouthwash products have shown potential to decrease the viral load in the oral cavity [151]. In the S3-level evidence-based treatment guidelines provided by the European Federation of Periodontology (EFP) in 2020, the use of mouthwash products is recommended as adjunctive agents in the treatment of stages I-III periodontitis [152].
Considerations for Oral Healthcare in View of this Hypothesis
Despite the scarcity of clinical evidence for in vivo viral inactivation, well-established mouthwashes containing specific ingredients, which inactivate SARS-CoV-2 in vitro, could potentially help mitigate transmission and decrease the risk of severe lung disease in COVID-19 [138,139]. These ingredients include:
- 0.05%-0.1% Cetylpyridinium Chloride (CPC): 15 ml for 30 seconds twice a day. In vitro and in vivo studies indicate that mouthwash products containing CPC are able to inactivate SARS-CoV-2 [137-140]. These products are generally considered to be safe, with staining of the tongue and teeth being rarely reported [153].
- 0.147% Ethyl lauroyl arginate (ELA): 20 ml for 30 seconds twice a day. In vitro results suggest virucidal activity of ELA against SARS-CoV-2 [138].
- 0.2%, 0.4% or 0.5% Povidone-Iodine (PVP-I): 10 ml for 30 seconds twice a day. The use of PVP-I is supported by in vitro [141-43] and in vivo studies [139,144-46]. In one clinical trial, the use of 1% PVP-I mouth rinse resulted in temporary thyroid dysfunction in 42% of COVID-19 patients, suggesting that lower concentrations should be preferred [144]. Contraindications: allergy, hyperthyroidism, thyroid dysfunction, pregnancy, lactation, and treatment with radioactive iodine [154,155].
The use of mouthwashes should not replace other daily oral hygiene measures. They should be used after toothbrushing for limited periods due to potential side effects. Mouthwashes should never be swallowed. In the context of the pandemic, it would seem logical to use mouthwash products both before and after social interactions, but clinical trials are required to answer the critical question of the potential effect of these products as a means of reducing the risk of transmission between individuals. Clinical trials are also required to specifically address the potential for mouthwashes to mitigate the development of COVID-19 lung disease, and hence the severest form of the disease.
Conclusion
The nasal and oral cavities are entry points into the body for microbial pathogens. The oral cavity provides favorable conditions for viral replication, with saliva functioning as a reservoir for SARS-CoV-2. Cells in different oral niches express receptors that make them potential targets for viral infection. The gingival sulcus in healthy patients can allow the entrance of viral particles into gingival capillaries due to the permeability of the sulcular epithelium. In periodontitis, the entrance of the virus into the circulation could be facilitated by micro-ulcerations in the pocket epithelium. Poor oral hygiene and dental plaque accumulation may further intensify this pathway. From gingival capillaries, the virus could reach the lungs via a vascular route, where it would trigger the known main pathological driver of disease, that of immunothrombosis. This proposed mechanism of transmission of the virus from the oral cavity to the lungs may explain both the radiological appearances and the clinical variability of the disease. Although to an extent the passage of the virus across the mucous membranes of the nasal passage and mouth may occur in healthy individuals, the presence of poor oral health could act as a risk factor to identify individuals more likely to develop COVID-19 lung disease, or those who might progress to severe disease leading to intensive care admission, mechanical ventilation, or death. The co-morbid presence of periodontitis has been shown to represent an independent risk factor for other systemic inflammatory diseases that are characterized by hyper-inflammation and oxidative stress [67,103,105].
Given that – i) saliva is a reservoir of the SARS-CoV-2 virus; ii) high salivary viral load is a better predictor of poor outcome than patient age; iii) the virus initially interacts with the upper respiratory airways; iv) the lower respiratory tract is not dominantly involved radiologically until the airways are compromised secondary to the vascular disease; v) the pulmonary vessels are dominantly affected radiologically; and vi) the radiological manifestations align with the model of viral binding with pulmonary vessel endothelial ACE2 receptors and subsequent processes – it seems compelling that a breach in the mucosal defenses of the mouth could facilitate transmission of SARS-CoV-2 to the lungs via a hematogenous route.
Studies are urgently required to confirm or refute this hypothesis. Determining viral load within blood samples taken simultaneously from the jugular vein and a peripheral site is suggested here as a possible means of corroborating the role of the proposed anatomical pathway. This method, by which the virus might be caught ‘red-handed’ as it passes down the jugular veins, has recently been offered with interest to laboratories and research teams in the UK, but is also offered here as an open proposal to researchers worldwide. It is not known if viable infectious virus could be transported by this route. This will need to be examined in future studies using a combination of electron microscopy to identify whether virus particles are being carried within or on particular cell types, for example macrophages, and functional assays to determine whether infectious virus can be rescued from these blood cells. If a differential viral load between samples taken simultaneously from a jugular vein and a peripheral site was established, then further corroboration of the theory could be gained by matching jugular viral load with salivary viral load, the severity of periodontitis, and post challenge following use of specific mouthwashes or other oral hygiene measures.
Until proven or refuted, daily oral hygiene and other measures for plaque control, together with oral healthcare should be prioritized for the general public, since these measures not only improve oral health and wellbeing but could also be potentially lifesaving in the context of the pandemic. There is already a precedent for employing simple oral hygiene measures to improve outcomes in patients with pneumonia, and it seems unlikely that oral hygiene measures will cause harm, as long as the manufacturer’s instructions are followed carefully.
In consideration of people in areas of the world where specific mouthwashes may not be available or affordable, there may be even simpler measures to consider. A recent study reported that saltwater rinsing can reduce gingival inflammation [156], and even mouth rinsing with boiled water (which has been allowed to cool) has shown positive effects on plaque and oral mucosa in hospitalized elderly patients [157]. This suggests that simple measures can help decrease the salivary viral load in areas of the world where mouthwash products are not readily available. It is sincerely hoped that, if proven correct, this concept provides a rationale for the use of oral healthcare measures which are cheap (or even free) and are available worldwide, and that these measures may help prevent the development of lung disease, mitigate deterioration to severe COVID-19, and reduce mortality.
References
- Hodges G, Pallisgaard J, Schjerning Olsen AM, McGettigan P, et al. (2020) Association between biomarkers and COVID-19 severity and mortality: A nationwide Danish cohortstudy. BMJ Open. 10(12):e041295.
- Chen M, Shen W, Rowan NR, Kulaga H, Hillel A, et al. (2020) Elevated ACE-2 expression in the olfactory neuroepithelium: Implications for anosmia and upper respiratory SARS-CoV-2 entry and replication. Eur Respir J. 2020.
- Sungnak W, Huang N, Bécavin C, Berg M, Queen R, et al. (2021) SARS-CoV-2 entry factors are highly expressed in nasal epithelial cells together with innate immune genes. Nat Med. 26(5):681-7.
- Lang M, Som A, Carey D, Reid N, Mendoza DP, et al. (2020) Pulmonary Vascular Manifestations of COVID-19 Pneumonia. Radiol Cardiothorac Imaging, 2(3):e200277.
- Oudkerk M, Kuijpers D, Oudkerk SF, van Beek EJ. (2020) The vascular nature of COVID-19. Br J Radiol. 93(1113):20200718.
- Oudkerk M, Buller HR, Kuijpers D, van Es N, Oudkerk SF, et al. (2021) Diagnosis, prevention, and treatment of thromboembolic complications in COVID-19: Report of the national institute for public health of the Netherlands. Radiology. 297(1):E216-22.
- Nemec SF, Bankier AA, Eisenberg RL. (2021) Lower lobe-predominant diseases of the lung. Am J Roentgenol. 200(4):712-28.
- Patwa A, Shah A. (2015) Anatomy and physiology of respiratory system relevant to anaesthesia. Indian J Anaesth. 2015 Sep; 59(9): 533-41.
- Luo L, Luo Z, Jia Y, Zhou C, He J, et al. (2020) CT differential diagnosis of COVID-19 and non-COVID-19 in symptomatic suspects: A practical scoring method. BMC Pulm Med. 20(1):129.
- Ufuk F, Savaş R. (2021) Chest CT features of the novel coronavirus disease (COVID-19). Turk J Med Sci. 50(4):664-78.
- Eddy RL, Sin DD. (2020) Computed Tomography Vascular Tree-in-Bud: A Novel Prognostic Imaging Biomarker in COVID-19? Am J Respir Crit Care Med. 202(5):642-44.
- Patel B V, Arachchillage DJ, Ridge CA, Bianchi P, Doyle JF, et al. (2020) lmonary angiopathy in severe COVID-19: Physiologic, imaging, and hematologic observations. Am J Respir Crit Care Med. 202(5):690-9.
- Ridge CA, Desai SR, Jeyin N, Mahon C, Lother DL, et al. (2021) Dual-Energy CT Pulmonary Angiography (DECTPA) Quantifies Vasculopathy in Severe COVID-19 Pneumonia. Radiol Cardiothorac Imaging. 2(5):e200428.
- van Dam LF, Kroft LJM, van der Wal LI, Cannegieter SC, Eikenboom J, et al. (2020) Clinical and computed tomography characteristics of COVID-19 associated acute pulmonary embolism: A different phenotype of thrombotic disease? Thromb Res. 193:86-9.
- Bernheim A, Mei X, Huang M, Yang Y, Fayad ZA, et al. (2020) Chest CT findings in coronavirus disease 2019 (COVID-19): Relationship to duration of infection. Radiology. 295:685-91.
- Shi H, Han X, Jiang N, Cao Y, Alwalid O, et al. (2020) Radiological findings from 81 patients with COVID-19 pneumonia in Wuhan, China: a descriptive study. Lancet Infect Dis. 20:425-34.
- Nicolai L, Leunig A, Brambs S, Kaiser R, Weinberger T, et al. (2020) Immunothrombotic dysregulation in COVID-19 pneumonia is associated with respiratory failure and coagulopathy. Circulation. 142(12):1176-1189.
- Nakazawa D, Ishizu A. (2020) Immunothrombosis in severe COVID-19. EBioMedicine. 59:102942.
- Kirchner J, Obermann A, Stuckradt S, Tushaus C, Goltz J, et al. (2015) Lung infarction following pulmonary embolism: A comparative study on clinical conditions and CT findings to identify predisposing factors. Rofo. 187(6):440-4.
- Martini K, Blüthgen C, Walter JE, Linh Nguyen-Kim TD, Thienemann F, et al. (2020) Patterns of organizing pneumonia and microinfarcts as surrogate for endothelial disruption and microangiopathic thromboembolic events in patients with coronavirus disease 2019. PLoS One. 15(10):e0240078
- Lax SF, Skok K, Zechner P, Kessler HH, Kaufmann N, et al. (2020) Pulmonary Arterial Thrombosis in COVID-19 With Fatal Outcome : Results From a Prospective, Single-Center, Clinicopathologic Case Series. Ann Intern Med. 14:M20-2566.
- Huertas A, Montani D, Savale L, Pichon J, Tu L, et al. (2020) Endothelial cell dysfunction: A major player in SARS-CoV-2 infection (COVID-19)? Eur Respir J. 56(1):2001634.
- Varga Z, Flammer AJ, Steiger P, Haberecker M, Andermatt R, et al.(2020) Endothelial cell infection and endotheliitis in COVID-19. Lancet. 395(10234):1417-18.
- Fox SE, Akmatbekov A, Harbert JL, Li G, Quincy Brown J, et al. Pulmonary and cardiac pathology in African American patients with COVID-19: an autopsy series from New Orleans. Lancet Respir Med. 8(7):681-86.
- McGonagle D, Bridgewood C, Ramanan AV, Meaney JFM, Watad A. (2021) COVID-19 vasculitis and novel vasculitis mimics. Lancet Rheumatol. 3(3):e224-e233.
- Ackermann M, Verleden SE, Kuehnel M, Haverich A, Welte T, et al. (2020) Pulmonary Vascular Endothelialitis, Thrombosis, and Angiogenesis in Covid-19. N Engl J Med. 383(2):120-8.
- Samavati L, Uhal BD. (2020) ACE2, Much More Than Just a Receptor for SARS-COV-2. Front Cell Infect Microbiol. 10:317.
- Murgolo N, Therien AG, Howell B, Klein D, Koeplinger K, et al. (2021) SARS-CoV-2 tropism, entry, replication, and propagation: Considerations for drug discovery and development. PLoS Pathog. 17(2):e1009225.
- Huang N, Perez P, Kato T, Mikami Y, Okuda K, et al. (2020) Integrated single-cell atlases reveal an oral SARS-CoV-2 infection and transmission axis. medRxiv.
- Sakaguchi W, Kubota N, Shimizu T, Saruta J, Fuchida S, et al. (2020) Existence of SARS-CoV-2 entry molecules in the oral cavity. Int J Mol Sci. 21(17):6000.
- Badran Z, Gaudin A, Struillou X, Amador G, Soueidan A. (2020) Periodontal pockets: A potential reservoir for SARS-CoV-2? Med Hypotheses. 143:109907.
- Gupta S, Mohindra R, Chauhan PK, Singla V, Goyal K, et al. (2021) SARS-CoV-2 Detection in Gingival Crevicular Fluid. J Dent Res. 100(2):187-193.
- Fernandes Matuck B, Dolhnikoff M, Maia GVA, Isaac Sendyk D, Zarpellon A, et al. (2020) Periodontal tissues are targets for Sars-Cov-2: a post-mortem study. J Oral Microbiol. 13(1):1848135.
- Huang N, Pérez P, Kato T, Mikami Y, Okuda K, et al. (2021) SARS-CoV-2 infection of the oral cavity and saliva. Nat Med.
- Silva J, Lucas C, Sundaram M, Israelow B, Wong P, et al. Saliva viral load is a dynamic unifying correlate of COVID-19 severity and mortality. Prepr Serv Heal.
- https://www.cdc.gov/coronavirus/2019-ncov/need-extra-precautions/older-adults.html
- Pallos D, Ruivo GF, Ferrari-Junior SH, Pannuti CS, Perozini C, et al. (2020) Periodontal disease and detection of human herpesviruses in saliva and gingival crevicular fluid of chronic kidney disease patients. J Periodontol. 91(9):1139-47.
- Contreras A, Nowzari H, Slots J. Herpesviruses in periodontal pocket and gingival tissue specimens. Oral Microbiol Immunol. 15(1):15-8.
- Musso D, Roche C, Nhan TX, Robin E, Teissier A, et al. (2015) Detection of Zika virus in saliva. J Clin Virol.68:53-5.
- Matičić M, Poljak M, Kramar B, Tomažić J, Vidmar L, et al. (2000) Proviral HIV-1 DNA in gingival crevicular fluid of HIV-1-infected patients in various stages of HIV disease. J Dent Res. 79(7):1496-501.
- Parra B, Slots J. (1996) Detection of human viruses in periodontal pockets using polymerase chain reaction. Oral Microbiol Immunol [Internet]. 11(5):289-93.
- Hujoel PP, White BA, García RI, Listgarten MA. (2001) The dentogingival epithelial surface area revisited. J Periodontal Res. 6(1):48-55.
- Wright HJ, Matthews JB, Chapple ILC, Ling-Mountford N, Cooper PR. (2008) Periodontitis Associates with a Type 1 IFN Signature in Peripheral Blood Neutrophils. J Immunol. 181(8):5775-84.
- Sampson V, Kamona N, Sampson A. (2020) Could there be a link between oral hygiene and the severity of SARS-CoV-2 infections? Br Dent J. 228(12):971-5.
- Bao L, Zhang C, Dong J, Zhao L, Li Y, Sun J. (2020) Oral Microbiome and SARS-CoV-2: Beware of Lung Co-infection. Front Microbiol. 11:1840.
- Marouf N, Cai W, Said KN, Daas H, Diab H, et al. (2021) Association between periodontitis and severity of COVID‐19 infection: A case–control study. J Clin Periodontol. 2021.
- Bosshardt DD. (2018) The periodontal pocket: pathogenesis, histopathology and consequences. Periodontology 2000. 76(1):43-50.
- Shimono M, Ishikawa T, Enokiya Y, Muramatsu T, Matsuzaka KI, et al. (2003) Biological characteristics of the junctional epithelium. J Electron Microsc. 52(6):627-39.
- Ito H-O. (2006) Infective endocarditis and dental procedures: evidence, pathogenesis, and prevention. J Med Investig. 53(3-4):189-98.
- Foster KJ, Jauregui E, Tajudeen B, Bishehsari F, Mahdavinia M. (2020) Smell loss is a prognostic factor for lower severity of coronavirus disease 2019. Ann Allergy, Asthma Immunol. 125(4):481-3.
- Slots J. (2007) Herpesviral–bacterial synergy in the pathogenesis of human periodontitis. Curr Opin Infect Dis. 20(3):278-83.
- Slots J. (2000) Herpesviral-bacterial interactions in periodontal diseases. Periodontol 2000. 52(1):117-40.
- Slots J. (2000) Herpesviruses in periodontal diseases. Periodontology 2000. 38:33-62.
- Mizgerd JP. (2008) Acute Lower Respiratory Tract Infection. N Engl J Med. 358(7):716-27.
- Bhatraju PK, Ghassemieh BJ, Nichols M, Kim R, Jerome KR, et al. (2020) Covid-19 in Critically Ill Patients in the Seattle Region- Case Series. N Engl J Med. 382(21):2012-22.
- Vincent-Bugnas S, Vitale S, Mouline CC, Khaali W, Charbit Y, et al. (2013) EBV Infection Is Common in Gingival Epithelial Cells of the Periodontium and Worsens during Chronic Periodontitis. Glogauer M, editor. PLoS One. 8(12):e80336.
- Imai K, Ochiai K, Okamoto T. (2009) Reactivation of Latent HIV-1 Infection by the Periodontopathic Bacterium Porphyromonas gingivalis Involves Histone Modification. J Immunol. 182(6):3688-95.
- Imai K, Inoue H, Tamura M, Cueno ME, Inoue H, et al. The periodontal pathogen Porphyromonas gingivalis induces the Epstein-Barr virus lytic switch transactivator ZEBRA by histone modification. Biochimie. 94(3):839-46.
- Stern J, Shai E, Zaks B, Halabi A, Houri-Haddad Y, et al. (2004) Reduced expression of gamma interferon in serum and marked lymphoid depletion induced by Porphyromonas gingivalis increase murine morbidity and mortality due to cytomegalovirus infection. Infect Immun. 72(10):5791-8.
- Pan W, Wang Q, Chen Q. (2019) The cytokine network involved in the host immune response to periodontitis. Int J Oral Sci. 11:1-13.
- Gemmell E, Marshall RI, Seymour GJ. (1997) Cytokines and prostaglandins in immune homeostasis and tissue destruction in periodontal disease. Periodontol 2000. 14(1):112-43.
- Cardoso EM, Reis C, Manzanares-Céspedes MC. Chronic periodontitis, inflammatory cytokines, and interrelationship with other chronic diseases. Postgrad Med. 130(1):98-104.
- Almaghlouth AA, Cionca N, Cancela JA, Décaillet F, Courvoisier DS, et al. (2014) Effect of periodontal treatment on peak serum levels of inflammatory markers. Clin Oral Investig. 18(9):2113-21.
- Gomes-Filho IS, Freitas Coelho JM, da Cruz SS, Passos JS, Teixeira de Freitas CO, et al. (2011) Chronic Periodontitis and C-Reactive Protein Levels. J Periodontol. 82(7):969-78.
- Genco RJ, Sanz M. (2020) Clinical and public health implications of periodontal and systemic diseases: An overview. Periodontology 2000. 83(1):7-13.
- Ling MR, Chapple ILC, Matthews JB. (2015) Peripheral blood neutrophil cytokine hyper-reactivity in chronic periodontitis. Innate Immun. 21(7):714-25.
- Sanz M, Ceriello A, Buysschaert M, Chapple I, Demmer RT, et al.(2018) Scientific evidence on the links between periodontal diseases and diabetes: Consensus report and guidelines of the joint workshop on periodontal diseases and diabetes by the International diabetes Federation and the European Federation of Periodontology. Diabetes Res Clin Pract. 137:231-41.
- Vidal F, Cordovil I, Figueredo CMS, Fischer RG. (2013) Non-surgical periodontal treatment reduces cardiovascular risk in refractory hypertensive patients: A pilot study. J Clin Periodontol. 40(7):681-7.
- Zhou SY, Duan XQ, Hu R, Ouyang XY. (2013) Effect of non-surgical periodontal therapy on serum levels of TNF-a, IL-6 and C-reactive protein in periodontitis subjects with stable coronary heart disease. Chin J Dent Res. 16(2):145-51.
- Higashi Y, Goto C, Hidaka T, Soga J, Nakamura S, et al. (2009) Oral infection-inflammatory pathway, periodontitis, is a risk factor for endothelial dysfunction in patients with coronary artery disease. Atherosclerosis. 206(2):604-10.
- Teeuw WJ, Slot DE, Susanto H, Gerdes VEA, Abbas F, et al. Treatment of periodontitis improves the atherosclerotic profile: A systematic review and meta-analysis. J Clin Periodontol. 41(1):70-9.
- Montenegro MM, Ribeiro IWJ, Kampits C, Saffi MAL, Furtado MV, et al. (2019) Randomized controlled trial of the effect of periodontal treatment on cardiovascular risk biomarkers in patients with stable coronary artery disease: Preliminary findings of 3 months. J Clin Periodontol. 46(3):321-31.
- Sanz M, Marco del Castillo A, Jepsen S, Gonzalez-Juanatey JR, D’Aiuto F, et al. (2020) Periodontitis and cardiovascular diseases: Consensus report. J Clin Periodontol. 47(3):268-88.
- Tonetti MS, D’Aiuto F, Nibali L. (2007) Treatment of periodontitis and endothelial function. N Engl J Med. 356(9):911-20.
- Coomes EA, Haghbayan H. (2020) Interleukin-6 in COVID-19: A Systematic Review and Meta-Analysis. Rev Med Virol. 26:e2141.
- Manson JJ, Crooks C, Naja M, Ledlie A, Goulden B, et al. (2020) COVID-19-associated hyperinflammation and escalation of patient care: a retrospective longitudinal cohort study. Lancet Rheumatol. 2(10):e594-602.
- Herold T, Jurinovic V, Arnreich C, Hellmuth JC, von Bergwelt-Baildon M, et al. (2020) Level of IL-6 predicts respiratory failure in hospitalized symptomatic COVID-19 patients. medRxiv.
- Zeng XT, Tu ML, Liu DY, Zheng D, Zhang J, et al. (2012) Periodontal Disease and Risk of Chronic Obstructive Pulmonary Disease: A Meta-Analysis of Observational Studies. PLoS One. 7(10).
- Scannapieco FA, Bush RB, Paju S. (2003) Associations between periodontal disease and risk for nosocomial bacterial pneumonia and chronic obstructive pulmonary disease. A systematic review. Ann Periodontol. 8(1):54-69.
- Sabharwal A, Gomes-Filho IS, Stellrecht E, Scannapieco FA. Role of periodontal therapy in management of common complex systemic diseases and conditions: An update. Periodontology 2000. 78(1):212-26.
- Holtfreter B, Richter S, Kocher T, Dörr M, Völzke H, et al. (2013) Periodontitis is related to lung volumes and airflow limitation: A cross-sectional study. Eur Respir J. 42(6):1524-35.
- Lee E, Lee SW. (2019) Prevalence of periodontitis and its association with reduced pulmonary function: Results from the Korean national health and nutrition examination survey. Medicina (Kaunas). 55(9):581.
- Peter KP, Mute BR, Doiphode SS, Bardapurkar SJ, Borkar MS, et al. (20130 Association Between Periodontal Disease and Chronic Obstructive Pulmonary Disease: A Reality or Just a Dogma? J Periodontol. 84(12):1717-23.
- Sapey E, Yonel Z, Edgar R, Parmar S, Hobbins S, et al. The clinical and inflammatory relationships between periodontitis and chronic obstructive pulmonary disease. J Clin Periodontol. 47(9):1040-52.
- Leung JM, Niikura M, Wei Tony Yang C, Sin DD. (2020) COVID-19 and COPD. Eur Respir J. 56(2):2002108.
- Alqahtani JS, Oyelade T, Aldhahir AM, Alghamdi SM, Almehmadi M, et al. (2020) Prevalence, severity and mortality associated with COPD and smoking in patients with COVID-19: A rapid systematic review and meta-analysis. PLoS ONE. 15(5):e0233147.
- Bellissimo-Rodrigues WT, Menegueti MG, Gaspar GG, Nicolini EA, Auxiliadora-Martins M, et al. (2014) Effectiveness of a Dental Care Intervention in the Prevention of Lower Respiratory Tract Nosocomial Infections among Intensive Care Patients: A Randomized Clinical Trial. Infect Control Hosp Epidemiol. 35(11):1342-8.
- Satheeshkumar PS, Papatheodorou S, Sonis S. (2020) Enhanced oral hygiene interventions as a risk mitigation strategy for the prevention of non-ventilator-associated pneumonia: a systematic review and meta-analysis. Br Dent J. 228(8):615-22.
- Scannapieco FA. (1999) Role of Oral Bacteria in Respiratory Infection. J Periodontol. 70(7):793-802.
- Muller F. (2015) Oral hygiene reduces the mortality from aspiration pneumonia in frail elders. J Dent Res. 94(3_suppl):14S-16S.
- Sjogren P, Nilsson E, Forsell M, Johansson O, Hoogstraate J. (2008) A systematic review of the preventive effect of oral hygiene on pneumonia and respiratory tract infection in elderly people in hospitals and nursing homes: Effect estimates and methodological quality of randomized controlled trials. J Am Geriatr Soc. 56(11):2124-30.
- Darby I. (2015) Periodontal considerations in older individuals. Aust Dent J. 60(S1):14-9.
- Mahase E. (2020) Covid-19: Why are age and obesity risk factors for serious disease? BMJ. 371:m4130.
- Peckham H, de Gruijter NM, Raine C, Radziszewska A, Ciurtin C, et al. (2020) Male sex identified by global COVID-19 meta-analysis as a risk factor for death and ITU admission. Nat Commun. 11(1):1-10.
- Ioannidou E. (2017) The sex and gender intersection in chronic periodontitis. Front Public Heal [Internet]. 5:189.
- Apicella M, Campopiano MC, Mantuano M, Mazoni L, Coppelli A, et al. (2020) COVID-19 in people with diabetes: understanding the reasons for worse. Lancet Diabetes Endocrinol. 8:782-92.
- Preshaw PM, Alba AL, Herrera D, Jepsen S, Konstantinidis A, et al. (2012) Periodontitis and diabetes: A two-way relationship. Diabetologia. 55(1):21-31.
- Nishiga M, Wang DW, Han Y, Lewis DB, Wu JC. (2020) COVID-19 and cardiovascular disease: from basic mechanisms to clinical perspectives. Nat Rev Cardiol. 17:543-58.
- Tonetti MS, Van Dyke TE. (2013) Periodontitis and atherosclerotic cardiovascular disease: consensus report of the Joint EFP/AAPWorkshop on Periodontitis and Systemic Diseases. J Periodontol. 84(4-s):S24-9.
- Martinez-Herrera M, Silvestre-Rangil J, Silvestre FJ. (2017) Association between obesity and periodontal disease. A systematic review of epidemiological studies and controlled clinical trials Med Oral Patol Oral Cir Bucal. 22(6):e708-e715.
- Si Y, Fan H, Song Y, Zhou X, Zhang J, Wang Z. (2012) Association Between Periodontitis and Chronic Obstructive Pulmonary Disease in a Chinese Population. J Periodontol. 83(10):1288-96.
- Zhao Q, Meng M, Kumar R, Wu Y, Huang J, et al. (2020) The impact of COPD and smoking history on the severity of COVID-19: A systemic review and meta-analysis. J Med Virol. 92(10):1915-21.
- Frydman A, Nowzari H. (2012) Down syndrome-associated periodontitis: a critical review of the literature. Compend Contin Educ Dent. 33(5):356-61.
- https://www.cdc.gov/coronavirus/2019-ncov/need-extra-precautions/people-with-medical-conditions.html#downsyndrome
- Eke PI, Dye BA, Wei L, Slade GD, Thornton-Evans GO, et al. (2015) Update on Prevalence of Periodontitis in Adults in the United States: NHANES 2009 to 2012. J Periodontol. 86(5):611-22.
- https://www.cdc.gov/coronavirus/2019-ncov/covid-data/investigations-discovery/hospitalization-death-by-race-ethnicity.html
- Pai GP, Dayakar MM, Shaila M, Dayakar A. (2012) Correlation between “aBO” blood group phenotypes and periodontal disease: Prevalence in south Kanara district, Karnataka state, India. J Indian Soc Periodontol. 16(4):519-23.
- Zhao J, Yang Y, Huang H, Li D, Gu D, Lu X, et al. (2020) Relationship between the ABO Blood Group and the COVID-19 Susceptibility. medRxiv.
- Sharma P, Fenton A, Dias IHK, Heaton B, Brown CLR, et al. (2020) Oxidative stress links periodontal inflammation and renal function. J Clin Periodontol. 48(3).
- Gansevoort RT, Hilbrands LB. (2020) CKD is a key risk factor for COVID-19 mortality. Nat Rev Nephrol. 16(12):705-06.
- Sharma P, Dietrich T, Ferro CJ, Cockwell P, Chapple ILC. (2016) Association between periodontitis and mortality in stages 3-5 chronic kidney disease: NHANES III and linked mortality study. J Clin Periodontol. 43(2):104-13.
- Ameer N, Palaparthi R, Neerudu M, Palakuru SK, Singam HR, et al. (2012) Oral hygiene and periodontal status of teenagers with special needs in the district of Nalgonda, India. J Indian Soc Periodontol. 16(3):421-5.
- Louapre C, Collongues N, Stankoff B, Giannesini C, Papeix C, et al. (2020) Clinical Characteristics and Outcomes in Patients with Coronavirus Disease 2019 and Multiple Sclerosis. JAMA Neurol. 77(9):1079-88.
- Abbayya K, Puthanakar NY, Naduwinmani S, Chidambar YS. (2015) Association between periodontitis and alzheimer’s disease. N Am J Med Sci. 7(6):241-6.
- Wang Q, Davis PB, Gurney ME, Xu R. (2021) COVID‐19 and dementia: Analyses of risk, disparity, and outcomes from electronic health records in the US. Alzheimer’s Dement.
- Tomar SL, Asma S. (2000) Smoking-Attributable Periodontitis in the United States: Findings From NHANES III. J Periodontol. 71(5):743-51.
- Reddy RK, Charles WN, Sklavounos A, Dutt A, Seed PT, et al. (2021) The effect of smoking on COVID‐19 severity: A systematic review and meta‐analysis. J Med Virol. 93(2):1045-56.
- Rossato M, Russo L, Mazzocut S, Di Vincenzo A, Fioretto P, et al. (2020) Current smoking is not associated with COVID-19. Eur Resp J. 55(6).
- Wong CM, Yang L, Chan KP, Chan WM, Song L, et al. (2013) Cigarette smoking as a risk factor for influenza-associated mortality: Evidence from an elderly cohort. Influenza Other Respir Viruses. 7(4):531-9.
- Polosa R, Caci G. (2020) COVID-19: counter-intuitive data on smoking prevalence and therapeutic implications for nicotine. Intern Emerg Med. Springer. 15:853-6.
- Farsalinos K, Barbouni A, Niaura R. (2020) Systematic review of the prevalence of current smoking among hospitalized COVID-19 patients in China: could nicotine be a therapeutic option? Intern Emerg Med. 15(5):845-52.
- Shields AM, Faustini SE, Kristunas CA, Cook AM, Backhouse C, et al. (2021) Longitudinal protection following natural SARS-CoV-2 infection and early vaccine responses: insights from a cohort of community based dental health care professionals. medRxiv.
- Meenawat A, Govila V, Goel S, Verma S, Punn K, et al. (2015) Evaluation of the effect of nicotine and metabolites on the periodontal status and the mRNA expression of interleukin-1β in smokers with chronic periodontitis. J Indian Soc Periodontol. 19(4):381-87.
- Naderi NJ, Semyari H, Elahinia Z. (2015) The impact of smoking on gingiva: A histopathological study. Iran J Pathol. 10(3):214-20.
- Dietrich T, Bernimoulin J-P, Glynn RJ. (2004) The Effect of Cigareté Smoking on Gingival Bleeding. J Periodontol. 75(1):16-22.
- Hopkinson NS, Rossi N, El-Sayed-moustafa J, Laverty AA, Quint JK, et al. (2021) Current smoking and COVID-19 risk: Results from a population symptom app in over 2.4 million people. Thorax.
- Parasher A. (2020) COVID-19: Current understanding of its pathophysiology, clinical presentation and treatment. Postgrad Med J.
- Hikmet F, Mear L, Edvinsson A, Micke P, Uhlen M, et al. (2020) The protein expression profile of ACE2 in human tissues. Mol Syst Biol. 16(7).
- Varga Z, Flammer AJ, Steiger P, Haberecker M, Andermatt R, et al. (2020) Endothelial cell infection and endotheliitis in COVID-19. Vol. 395, The Lancet. Lancet Publishing Group. 395(10234):1417-8.
- Beyerstedt S, Casaro EB, Rangel EB. (2021) COVID-19: angiotensin-converting enzyme 2 (ACE2) expression and tissue susceptibility to SARS-CoV-2 infection. Eur J Clin Microbiol Infect Dis. Springer Science and Business Media Deutschland GmbH. Pp: 1-5.
- Kumar A, Narayan RK, Kumari C, Faiq MA, Kulandhasamy M, et al. (2020) SARS-CoV-2 cell entry receptor ACE2 mediated endothelial dysfunction leads to vascular thrombosis in COVID-19 patients. Med Hypotheses. 145:110320.
- Nakazawa D, Ishizu A. (2020) Immunothrombosis in severe COVID-19. EBioMedicine. Elsevier. 59.
- Senchenkova1 EY, Russell J, Vital SA, Yildirim1 A, Orr AW, et al. (2018) A critical role for both CD40 and VLA5 in angiotensin Il-mediated thrombosis and inflammation. FASEB J. 32(6):3448-56.
- Lloyd-Jones G, Oudkerk M. (2021) COVID-19: angiotensin II in development of lung immunothrombosis and vasculitis mimics. Lancet Rheumatol.
- Wolfel R, Corman VM, Guggemos W, Seilmaier M, Zange S, et al. (2020) Virological assessment of hospitalized patients with COVID-2019. Nature. 581(7809):465-9.
- Bermejo-Martin JF, Gonzalez-Rivera M, Almansa R, Micheloud D, Tedim AP, et al. (2020) Viral RNA load in plasma is associated with critical illness and a dysregulated host response in COVID-19. Crit Care. 24(1).
- Komine A, Yamaguchi E, Okamoto N, Yamamoto K. (2021) Virucidal activity of oral care products against SARS-CoV-2 in vitro. J Oral Maxillofac Surg Med Pathol.
- Statkute E, Rubina A, O’Donnell VB, Thomas DW, Stanton RJ. (2020) Brief report: The virucidal efficacy of oral rinse components against SARS-CoV-2 in vitro. bioRxiv.
- Seneviratne CJ, Balan P, Ko KKK, Udawatte NS, Lai D, et al. (2020) Efficacy of commercial mouth-rinses on SARS-CoV-2 viral load in saliva: randomized control trial in Singapore. Infection. 49(2).
- Green A, Roberts G, Tobery T, Vincent C, Barili M, et al. (2020) In vitro assessment of the virucidal activity of four mouthwashes containing Cetylpyridinium Chloride, ethanol, zinc and a mix of enzyme and proteins against a human coronavirus. bioRxiv.
- Pelletier JS, Tessema B, Frank S, Westover JB, Brown SM, et al. (2021) Efficacy of Povidone-Iodine Nasal and Oral Antiseptic Preparations Against Severe Acute Respiratory Syndrome-Coronavirus 2 (SARS-CoV-2). Ear, Nose Throat J. 100(2_suppl):192S-196S.
- Bidra AS, Pelletier JS, Westover JB, Frank S, Brown SM, et al. (2020) Rapid In-Vitro Inactivation of Severe Acute Respiratory Syndrome Coronavirus 2 (SARS-CoV-2) Using Povidone-Iodine Oral Antiseptic Rinse. J Prosthodont. 29(6):529-33.
- Anderson DE, Sivalingam V, Kang AEZ, Ananthanarayanan A, Arumugam H, et al. (2020) Povidone-Iodine Demonstrates Rapid In Vitro Virucidal Activity Against SARS-CoV-2, The Virus Causing COVID-19 Disease. Infect Dis Ther. 9(3):669-75.
- Guenezan J, Garcia M, Strasters D, Jousselin C, Leveque N, et al. (2021) Povidone Iodine Mouthwash, Gargle, and Nasal Spray to Reduce Nasopharyngeal Viral Load in Patients with COVID-19: A Randomized Clinical Trial [Internet]. JAMA Otolaryngology-Head and Neck Surgery. JAMA. Pp. E1-2.
- Martinez Lamas L, Diz Dios P, Perez Rodriguez MT, Del Campo P, Cabrera Alvargonzalez JJ, et al. (2020) Is povidone-iodine mouthwash effective against SARS-CoV-2? First in vivo tests. Oral Dis.
- Mohamed NA, Baharom N, Wan Sulaiman WS, Rashid ZZ, Ken WK, et al. (2020) Early viral Clearance among COVID-19 patients when gargling with povidone-iodine and essential oils- A clinical trial. medRxiv.
- Kirk-Bayley J, Challacombe S, Sunkaraneni V, Combes J. (2020) The Use of Povidone Iodine Nasal Spray and Mouthwash During the Current COVID-19 Pandemic May Protect Healthcare Workers and Reduce Cross Infection. SSRN Electron J.
- https://www.reuters.com/article/us-health-coronavirus-japan-gargling-idUSKCN2510PQ
- https://www.statista.com/statistics/1104709/coronavirus-deaths-worldwide-per-million-inhabitants/
- Chapple ILC, Van Der Weijden F, Doerfer C, Herrera D, et al. (2015) Primary prevention of periodontitis: Managing gingivitis. J Clinic Periodont. Blackwell Munksgaard. 42: S71-6.
- O’Donnell VB, Thomas D, Stanton R, Maillard J-Y, Murphy RC, et al. (2020) Potential Role of Oral Rinses Targeting the Viral Lipid Envelope in SARS-CoV-2 Infection. Function.
- Sanz M, Herrera D, Kebschull M, Chapple I, Jepsen S, et al. (2020) Treatment of stage I-III periodontitis-The EFP S3 level clinical practice guideline. J Clin Periodontol. 47(S22):4-60.
- Stookey GK, Beiswanger B, Mau M, Isaacs RL, Witt JJ, et al. (2005) A 6-month clinical study assessing the safety and efficacy of two cetylpyridinium chloride mouthrinses. Am J Dent. 18(SPEC. ISS.):24A-28A.
- Chopra diti, Sivaraman K, Radhakrishnan R, Balakrishnan D, Narayana A. (2021) Can povidone Iodine gargle/mouthrinse inactivate SARS-CoV-2 and decrease the risk of nosocomial and community transmission during the COVID-19 pandemic? An evidence-based update. Jpn Dent Sci Rev. 57:39.
- Frank S, Capriotti J, Brown SM, Tessema B. (2020) Povidone-Iodine Use in Sinonasal and Oral Cavities: A Review of Safety in the COVID-19 Era. Ear Nose Throat J. SAGE Publications Ltd. 99:586-93.
- Collins JR, Veras K, Hernandez M, Hou W, Hong H, et al. (2021) Anti-inflammatory effect of salt water and chlorhexidine 0.12% mouthrinse after periodontal surgery: a randomized prospective clinical study. Clin Oral Investig. 1-9.
- Chen SC, Weng LC, Tsai SC, Wang SM, Han HM. (2019) Effectiveness of Oral Rinsing Solutions on Mucus, Odor, and Plaque in the Hospitalized Elderly in Taiwan. Clin Nurs Res. 28(6):762