Graphene and Stem Cells: The Quiet Revolution in Bone Tissue Engineering
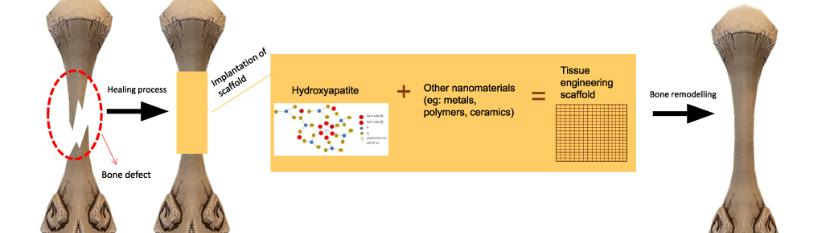
Ygor Gonçalves Felix de Mattos1*, Helena Coutinho Geiger Campos1*, Roger Borges1*, Guilherme FB Lenz E Silva2* and Daniela Franco Bueno1,2*
1Faculdade Israelita de Ciências da Saúde Albert Einstein (FICSAE), São Paulo, São Paulo, Brazil
2Engenharia Metalúrgica e de Materiais (POLI-USP), São Paulo, Brazil
*Corresponding author: Daniela Franco Bueno, Faculdade Israelita de Ciências da Saúde Albert Einstein (FICSAE), São Paulo, São Paulo, Brazil
Citation: Felix de Mattos YG, Campos HCG, Borges, Lenz E Silva GFB, Bueno DF . (2024) Graphene and Stem Cells: The Quiet Revolution in Bone Tissue Engineering. J Stem Cell Res. 5(1):1-8.
Received: August 22, 2024 | Published: September 15, 2024
Copyright© 2024 genesis pub by Felix de Mattos YG, et al. CC BY-NC-ND 4.0 DEED. This is an open-access article distributedunder the terms of the Creative Commons Attribution-NonCommercial-No Derivatives 4.0 International License.,This allows others distribute, remix, tweak, and build upon the work, even commercially, as long as they credit the authors for the original creation.
Introduction
Many problems related to bone tissue repair can be better addressed through a multidisciplinary approach to correcting bone defects. As a result, bone tissue bioengineering has become a reality in our time.
Tissue engineering is an interdisciplinary field that combines principles of biology, medicine, engineering, and materials science to develop biological substitutes that restore, maintain, or improve the function of damaged tissues and organs. This innovative field aims not only to replace damaged parts of the body but also to stimulate the body to regenerate its own tissues through biocompatible scaffolds, stem cells, and biochemical signals that mimic the natural environment of the tissues. Tissue engineering offers a promising prospect for treating various diseases and conditions, ranging from skin regeneration to burns, cartilage and bone reconstruction, and even entire organs [1].
The development of new strategies for bone regeneration remains a challenge for professionals such as dentists, orthopedic, and craniofacial surgeons, as they still encounter difficulties, for example, in obtaining autogenous bone for the reconstruction of large bone defects and in the acceptance of heterologous grafts in the body due to immunomodulatory factors, which vary from one organism to another. It is important to emphasize that the process of bone tissue regeneration/repair occurs through the sequential stages of inflammation, repair, and remodeling, involving multiple signaling pathways that act in coordination within the bone. Furthermore, for successful regeneration to occur, the processes of osteoinduction and osteoconduction must take place at the site of the bone defect to enable its repair. [4].
In this context, bone tissue engineering has gained momentum in the development of bones for various pathologies, as it involves the use of cells combined with different types of biomaterials that promote bone tissue regeneration [5].
The association of mesenchymal stem cells (mesenchymal stromal cells) with organic or inorganic biomaterials has been widely described in the literature and may lead to bone tissue regeneration in different degrees depending on the type of biomaterial (scaffold) selected for use in bone tissue engineering [6].
This opens a door for the use of graphene in the composition of the scaffold, which is considered revolutionary, since it has anti-inflammatory properties, is able to eliminate reactive oxygen species (ROS) and has high synergistic potential of conductivity [7]. Being able, then, to create an excellent platform for antimicrobial behavior and improvement related to the biocompatibility of the material and organism relationship. In addition to enabling the creation of two- or three-dimensional structures with varying porosities, electrical conductivity, surface area, surface defects, functionalization/degree of oxidation, and mechanical strength, capable of mimicking and supporting cellular growth and differentiation processes.
It is important to note that the accelerated osteogenesis of hMSCs in the presence of graphene was achieved without the addition of extra growth factors, and the rate of acceleration was comparable to that achieved with typical growth factors. This finding is significant because the physical characteristics of graphene and silicon dioxide that regulate the osteogenesis of hMSCs, such as stiffness, roughness, and topography, do not differ substantially [8].
It is essential to understand the chemical properties of graphene, so its cellular interaction with mesenchymal stem cells is better understood. To unravel the molecular basis underlying the accelerated differentiation of MSCs, we investigated the binding interactions between substrates and chemical inducers. It was then established the possibility of graphene to serve as a pre-concentration platform for various growth factors and differentiation chemicals through its unique ability to bind π - π non-covalent. The interaction π - π is a particular type of scattering force of van der Waals forces, established between polycyclic unsaturated molecules. Graphene along with carbon nanotubes have the same hexatomic ring of carbon atoms and therefore can be spontaneously stacked by these chemical interactions. In this case, stable solutions of CNT/graphene would be formed in addition to each other, from which hybrid materials CNT/graphene could be prepared [9].
Certain osteogenic-inducing serum proteins were observed to accumulate favorably in the graphene and consequently improve adhesion, proliferation and osteogenic differentiation of stem cells. The adipogenic differentiation of hMSCs was significantly suppressed as insulin, an important regulator in fatty acid synthesis, denatured in graphene due to its strong interactions π - π with graphene [9].
It is important to note that the gold standard for bone grafting is the autogenous graft, which, despite its efficacy, has significant limitations, such as donor site morbidity and limited availability, thereby restricting its application in clinical practice depending on the size of the defect to be regenerated [6]. Consequently, the field of bone tissue engineering is gaining traction in the search for new biomaterials to associate with mesenchymal stem cells, drawing significant global research interest [7]. This field has the potential to overcome the disadvantages associated with the use of bone banks, further expanding the possibilities for regenerative medicine, particularly with the integration of graphene, and enhancing our understanding of the basic biology and pathogenesis of bone diseases [8,9].
The Relevance of Stem Cells
Over the last decade, scientists have turned their attention to new forms of treatment and tissue regeneration, welcoming tissue engineering as a method for seeking better conditions for patient recovery and also for performing clinical practice. Thus, tissue engineering, by advocating the regeneration of target tissue that has been damaged, associate’s biomaterials with cell types that can adapt to the needs of the host.
Mesenchymal stem cells or stromal stem cells can be obtained from a variety of tissues, such as umbilical cord blood, menstrual blood, bone marrow, adipose tissue, tooth pulp cells and liposuction tissue, among others [8,10].
In bone tissue engineering, the use of MSCs derived from facial tissues, such as dental pulp, palatal periosteum, orbicularis oris muscle, and levator veli palatini muscle, is extremely promising. These cells originate from the neural crest, exhibit unlimited proliferative potential, and possess superior osteogenic differentiation capabilities [11]. In addition, they can differentiate into different cell types, such as osteoblasts, chondroblasts, adipocytes and myoblasts, besides being able to modulate the immune response, facilitating the adaptation of the organism [12].
Even though MSCs can be predisposed to differentiate into various tissues, their association with a biomaterial (scaffold) that provides the necessary characteristics for the development of bone tissue remains a challenge. In this context, the combination of MSCs with graphene has garnered global attention, as graphene possesses a specific surface area and the ability to control pore size during scaffold development, allowing MSC penetration and promoting osteoconduction. Moreover, graphene exhibits chemical and biological flexibility, which are crucial for cellular functionality [13] (Figure 1).
Despite the global enthusiasm surrounding the discovery of graphene, there remain significant challenges, as some authors have reported the potential toxicity of graphene to cells and tissues, which has yet to be fully elucidated by the scientific community. However, most scientific studies highlight positive attributes of graphene for use in bone tissue engineering and drug development. These studies indicate that graphene exhibits high biocompatibility, potential for drug biodistribution, and the ability to control its biodegradation, thereby opening new avenues for the use of this biomaterial in scaffold fabrication [14].
While the combination of graphene with mesenchymal stem cells offers numerous advantages for MSC adaptation to target tissues, further studies are needed to fully ensure the safety of its application in human bone tissue engineering [14,15].
Recent Advances
Due to the fact that scientific research is always continuous, new windows of opportunity for the use of new drugs and methods are increasingly opening up for the resolution of bone problems affecting thousands of lives [10]. In light of this, the development of stem cell research, particularly in relation to its association with graphene, is being conducted with future expectations in regenerative medicine in mind.
Following this path, advances such as reepithelialization, angiogenesis and collagen maturation for skin healing were described by Zhao et al. Furthermore, it was demonstrated through morphological and molecular analysis that the differentiation of MSC through graphene oxide (GO) increases the absorption of serum proteins and induces adhesion and proliferation [16,17].
In order to prove the efficiency of graphene in promoting mesenchymal stem cell adhesion, a study conducted in 2019 by Niknam et al. compared the differentiation of stem cells into osteoblasts during 0, 7 and 14 days, demonstrating that in polycaprolactone (PCL) polymer scaffolds are able to induce the activity of alkaline phosphatase (ALP) which is an early osteoblastic differentiation marker, indicating that graphene can induce osteogenic differentiation [18].
It is common knowledge that results achieved in the laboratory can often not be directly translated into the clinic, however, the graphene scaffolds for proliferation of MSCs have brought new emerging application potential, revolutionizing regenerative medicine and the field of tissue engineering.
In 2022, our research group conducted a scoping review on the use of graphene for bone tissue engineering, including articles published over the last 12 years (from 2010 to 2022) that utilized graphene and its derivatives (graphene oxide and reduced graphene) in preclinical studies for bone tissue regeneration. Following the search protocol and the application of inclusion criteria, 77 studies were selected and evaluated by five blind researchers. Most of the selected studies involved composite materials associated with graphene and its derivatives, combined with natural and synthetic polymers, bioglass, and other substances. Although a variety of graphene materials were analyzed in these studies, all concluded that graphene, its derivatives, and their composites enhance bone repair processes, increasing osteoconductivity, osteoinductivity, new bone formation, and angiogenesis, thereby opening new opportunities for the development of innovative strategies in bone tissue engineering using graphene [19].
To better understand the interaction between graphene and MSCs, the following table lists exemplifies graphene-based nanomaterials (GBNs) used as scaffolds, along with the different cell types and their corresponding reactions (Table 1).
Author |
GBNs |
Types of cells or tissue |
Effect |
Reference |
Shie M, et al (2017) |
CS/graphene composite |
Stem cells from human bone marrow |
Cell-friendly and higher levels of graphene were more favourable to cell proliferation |
22 |
Wu D, et al. (2017) |
Starch nanofibres incorporated into GO |
Osteoblast cells (MG 63) |
Promoted minealization of calcium and phosphorus |
23 |
Shanmuga s, et al. (2019) |
Functionalized chitosan nanoparticle GO |
Osteosarcoma cells (Saos-2 and MG-63) |
Cells of the osteosarcoma loaded by composite nanoparticle were relerased in the controlled way at acid PH. |
24 |
Challenges and Considerations
The use of graphene for scaffold construction represents a promising advance in tissue engineering, opening new possibilities for the development of innovative treatments. Although there are still discussions around ethical and regulatory considerations, such as safety and efficacy, these challenges have been addressed in the literature to ensure that graphene can be used safely and effectively in future dental and medical applications.
Thus, as described by Tiwari et al. (2019), despite the promising potential of combining graphene-derived nanomaterials with mesenchymal stem cells (MSCs) for bone tissue engineering, there are still critical challenges to be overcome. One of the main bottlenecks involves a detailed understanding of how these nanomaterials interact with MSCs at the microscopic level [3]. This interaction demands improvement in terms of fixation properties, controlled release of bioactive factors and compatibility with cell membranes, which are essential to ensure the effectiveness of treatment. In addition, balancing the regenerative potential with the minimization of cytotoxicity remains a substantial challenge, which requires continuous investigations to optimize the biocompatibility of materials and maximize the clinical safety of therapeutic applications [19,20].
Although there are inherent challenges in the use of graphene and its derivatives in association with mesenchymal stem cells (MSCs) for bone tissue engineering, scientific evidence highlights the significant potential of this material in the biomedical field.
The unique two-dimensional structure of graphene, which initially generated concerns due to its specific chemical and physical properties, has been extensively studied, revealing substantial advantages for bone regeneration. Graphene and its derivatives, such as graphene oxide, have demonstrated exceptional capabilities to promote cell adhesion, proliferation and osteogenic differentiation of MSCs, which can be attributed to their high surface area and ease of chemical functionalization [21].
Although concerns about biodegradability and potential toxic effects have been raised, recent studies have addressed these challenges, showing that the functionalization of graphene can improve its biocompatibility and minimize cytotoxicity. In addition, the toxicity observed in macrophages and lung models can be modulated by changes in the graphene surface, which allow a more controlled interaction with biological systems. Thus, rather than limiting its use, these characteristics encourage the development of new strategies that take advantage of the unique properties of graphene, while ensuring the safety and effectiveness of the material [19,21].
These advances, coupled with the growing body of scientific evidence supporting the use of graphene in bone regeneration, reinforce its role as an innovative and promising component in tissue engineering, especially when associated with MSCs [22-24].
Future Perspectives
The use of graphene in scaffolds for MSC association holds significant promise, offering new perspectives for bone tissue engineering by reducing patient risks associated with heterologous grafts and eliminating donor site morbidity in autologous grafts.
Current clinical practice demands more effective therapeutic approaches, benefiting both patients and healthcare professionals, including dentists focused on alveolar tissue regeneration and orthopedic surgeons aiming for the reintegration of fractured bone structures.
Given the technological and scientific advancements, it is crucial to foster integrated collaboration between scientists, researchers, and biotechnological drug and material production industries. Such collaboration is vital for advancing regenerative medicine, paving the way for innovative treatments that can enhance patient quality of life and optimize clinical practices for healthcare professionals.
Acknowledgements
We would like to thank the Foundation for Research Support of the State of São Paulo (FAPESP – Fundação de Amparo a Pesquisa do Estado de São Paulo) for financially supporting the Graphene/Stem Cell Research (grant no 2020/12954-2).
Declaration of Conflicting Interests
The authors declare that the research was conducted in the absence of any commercial or financial relationships that could be construed as a potential conflict of interest.
Funding
The authors disclosed receipt of the following financial support for the research on Graphene and stem cells in Brazil: Fundação de Amparo a Pesquisa do Estado de São Paulo (FAPESP grant no 2020/12954-2).
References
- Langer R, Vacanti JP. (1993) Tissue Engineering. Science. 260(5110):920-6.
- Sharma P, Kumar P, Sharma R, Bhatt VD, Dhot PS, et al. (2019) Tissue Engineering; Current Status & Futuristic Scope. J Med Life. 12(3): 225-29.
- LI Y, Lu Y, Hou Y, Zhang Z, Chen M, et al. (2022) Polydopamine-mediated graphene oxide and nanohydroxyapatite-incorporated conductive scaffold with an immunomodulatory ability accelerates periodontal bone regeneration in diabetes. Bioact Mater. 18:213-27.
- Siddiqui AJ, Partridge NC. (2016) Physiological Bone Remodeling: Systemic Regulation and Growth Factor Involvement. Physiology (Bethesda). 31(3):233-45.
- Elgali I, Omar O, Christer D, Thomsen P, et al. (2017) Guided bone regeneration: materials and biological mechanisms revisited. Eur J Oral Sci. 125(5): 315-37.
- Ercal P, Pekozer GG. (2020) A Current Overview of Scaffold-Based Bone Regeneration Strategies with Dental Stem Cells. Adv Exp Med and Biol. 1288:61-85.
- Pandit S, Gaska K, Kadar R, Mijakovic I. (2020) Graphene‐Based Antimicrobial Biomedical Surfaces. Chemphyschem. 22(3):250-263.
- Pinheiro CCG, Compos HCG, Mattos YGFD, Bueno DF. (2023) Bone Regeneration Capacity through Microvesicles and Exosomes. J Stem Cell Res Dev Ther. 9:109
- Ishihara K, A. SAKAKURA. (2014) 5.10 Hetero-Diels–Alder Reactions. Elsevier eBooks, p. 409–465, 2014.
- Kolois G, Moodley Y. (2012) Introduction to Stem Cells and Regenerative Medicine. Respiration. 85(1):3-10
- Bueno DF, kabayashi GS, Pinheiro CCG, Tanikawa DYS, Amral CER, et al. Human levator veli palatini muscle: a novel source of mesenchymal stromal cells for use in the rehabilitation of patients with congenital craniofacial malformations. Stem Cell Res Ther. 11(1):501.
- Schmidt AH. (2021) Autologous bone graft: Is it still the gold standard? Injury. 2:18-22.
- Ding DC, Shyu WC, Lin SZ. (2011) Mesenchymal Stem Cells. Cell Transplant. 20(1):5–14.
- Liu JA, Cheubg M. (2016) Neural crest stem cells and their potential therapeutic applications. Developmental Biology. 419(2):199–16
- Kenry, Lee WC, Loh KP, Lim CT. (2018) When stem cells meet graphene: Opportunities and challenges in regenerative medicine. Biomaterials. 155:236–50.
- Ikram R, Shamsuddin SAA, Jan BM, Qadir MA, Kenanakis G, et al. (2022) Impact of Graphene Derivatives as Artificial Extracellular Matrices on Mesenchymal Stem Cells. Molecules. 27(2):379–79.
- Lazarev S, Uzhviyuk S, Rayev M, Timganova V, Bochkova M, et al. (2023) Interaction of Graphene Oxide Nanoparticles with Human Mesenchymal Stem Cells Visualized in the Cell-IQ System. Molecules. 28(10):4148–48.
- Zhao M, Shi J, Cai W, Liu K, Shen K, et al. (2021) Advances on Graphene-Based Nanomaterials and Mesenchymal Stem Cell-Derived Exosomes Applied in Cutaneous Wound Healing. Int J Nanomedicine. 16:2647– 65.
- Achoa GL, Mattos PA, Clements PA, Roca Y, Brooks Z, et al. (2023) A scoping review of graphene-based biomaterials for in vivo bone tissue engineering. J Biomater Appl. 38(3):313–50.
- Niknam Z, Zali H, Mansouri V, Tavirani MR, Omidi M. (2019) Morphological and Molecular Analysis of Osteoblasts Differentiated from Mesenchymal Stem Cells in Polycaprolactone/Magnesium Oxide/Graphene Oxide Scaffold.Int J Organ Transplant Med. 10(4):171-82
- Farag MM. (2023). Recent trends on biomaterials for tissue regeneration applications: review. J Material Sci. 58(2):527–58.
- Shie MY, Chiang WH, Chen WP, Liu WY, Chen YW. (2017) Synergistic acceleration in the osteogenic and angiogenic differentiation of human mesenchymal stem cells by calcium silicate–graphene composites. Mater Sci Eng C Mater Biol Appl. 73:726–35.
- Wu D, Samanta A, Srivastava RK, Hakkarainen M. (2017) Starch-Derived Nanographene Oxide Paves the Way for Electrospinnable and Bioactive Starch Scaffolds for Bone Tissue Engineering. Biomacromolecules. 18(5):1582–91.
- Saravanabhavan S, Rethinasabapathy M, Zsolt S, Kalambettu AB, Elumalai S, et al. (2019) Graphene oxide functionalized with chitosan based nanoparticles as a carrier of siRNA in regulating Bcl-2 expression on Saos-2 & MG-63 cancer cells and its inflammatory response on bone marrow derived cells from mice. Mater Sci Eng C Mater Biol Appl. 99:1459–68.