Current Limitations and The Future Promise of Stem Cell-Derived Organoids in Renal Cell Carcinoma Treatment
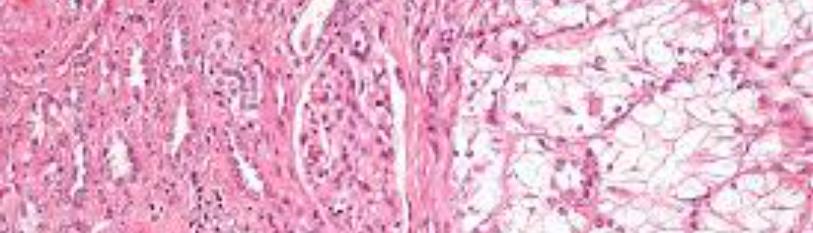
Dylan Monaghan1 and Vincent S. Gallicchio2*
1Department of Biological Sciences, College of Science, Clemson University, Clemson, SC, USA
2Department of Biological Sciences College of Science 122 Long Hall Clemson University Clemson, SC 29636
*Corresponding author: Vincent S Gallicchio, Department of Biological Sciences College of Science 122 Long Hall Clemson University Clemson, SC 29636.
Citation: Monaghan D, Gallicchio VS. (2023) Current Limitations and The Future Promise of Stem Cell-Derived Organoids in Renal Cell Carcinoma Treatment. J Stem Cell Res. 4(1):1-14.
Received: April 26, 2023 | Published: May 25, 2023
Copyright© 2023 genesis pub by Monaghan D, et al. CC BY NC-ND 4.0 DEED. This is an open-access article distributed under the terms of the Creative Commons Attribution-NonCommercial-No Derivatives 4.0 International License., This allows others distribute, remix, tweak, and build upon the work, even commercially, as long as they credit the authors for the original creation.
DOI: https://doi.org/10.52793/JSCR.2023.4(1)-47
Abstract
Renal Cell Carcinoma (RCC) is the most common type of urogenital cancer and is currently ranked as the sixth most common cancer among men and tenth among women. RCC is most often detected incidentally in individuals between the ages of 60 and 70 and possesses a high degree of heterogeneity with an extremely high risk of metastasis. Recently, the disease has become known to encompass differing histological subtypes and unique genetic and molecular modifications, even further complicating treatment options. The progression of the disease can affect the digestive, skeletal, circulatory, immune, nervous, and reproductive systems causing a wide range of symptoms including cough, chest and bone pain, nausea, shortness of breath, fever, seizures, and more. Due to the prevalence of unsuccessful treatments using customary methods, researchers have been eagerly searching to find innovative procedures to help patients suffering from RCC. With such a severe degree of heterogeneity, modern-day researchers have turned to stem cell-derived organoids as the potential hero in RCC cases. Pluripotent stem cells and, more recently, adult stem cells that differentiate into tumor organoids have provided promising platforms for organ/disease modelling, drug-induced nephrotoxicity studies, and personalized treatment plans for RCC patients. This review outlines the current research, progress, and limitations that stem cell-derived organoids have made in producing a curative treatment for RCC.
List of Abbreviations
CDC: Center for Disease Control and Prevention
RCC: Renal Cell Carcinoma
ccRCC: clear cell Renal Cell Carcinoma
pRCC: papillary Renal Cell Carcinoma
chRCC: chromophobe Renal Cell Carcinoma
LRN: Laparoscopic radial nephrectomy
ASC: adult stem cells
PSC: pluripotent stem cell
ESC: embryonic stem cell
iPSC: induced pluripotent stem cell
PDTO: patient-derived tumor organoid
TO: tumor organoid
hPSC: human pluripotent stem cell
TKI: tyrosine kinase inhibitors
PFS: progression free survival
FDA: food and drug administration
DNA: deoxyribonucleic acid
Keywords
Metastasis; Stem Cells; Organoids; Disease Modeling; Drug Treatment Therapy; Renal Cell Carcinoma
Introduction
What is Cancer?
Cancer is the leading cause of death worldwide. In 2020, the WHO reported about ten million deaths due to cancer across the world [1]. In 2022, roughly 1.9 million people were expected to be diagnosed with an estimated 609,360 to die in the United States alone [2]. The disease can develop in individuals of every age group but occurs most commonly in those middle-aged and older with a median age at diagnosis of 66 [3]. A 2019 screening from the CDC reported the following percentages of new cancers by age at diagnosis as follows [4] (Figure 1).
Figure 1: Rate of New Cancers by Age Group (Years), All Races and Ethnicities, Both Sexes [4].
There are over 100 types of cancer, of which each are usually named after the organs or tissues from which the cancers originated. The type may also be described based on the tissues that formed them. However, quite often, cancer cells break away from their origin and form new tumors in other parts of the body – a process called metastasis [5]. Cancer arises as a genetic disease resulting from changes in the genes that control the growth and division of cells. Despite the human body’s innate ability to eliminate cells with damaged DNA, this ability declines with age explaining the increased risk in later years of life [5]. Additionally, cancer often arises from the transformation of normal cells into tumor cells subsequently due to a combination of interactions between the person’s genome coupled with carcinogens such as physical, chemical, and biological carcinogens. Tobacco use, alcohol consumption, unhealthy diet, physical inactivity, and air pollution often play a major role as carcinogens in these interactions. Some cancers may even be caused by environmental factors such as UV radiation exposure in sunlight or viral/bacterial infections [1,7]. Genetic changes that induce cancer tend to affect two main classes of genes – oncogenes and tumor suppressor genes. Oncogenes function to transmit growth signals from the cell periphery to the nucleus. Altered growth signals effectively disturb these signaling function and cause the cells to become cancerous. Tumor suppressor genes act to have a reducing effect on cell growth but may be lost due to inactivating mutations or deletion of the gene. Ultimately, most cancers occur from a combination of multiple genetic changes in both oncogenes and tumor suppressor genes [8].
Renal Cell Carcinoma
In [9] concluded a worldwide estimate of about 400,000 new cases and 175,000 deaths due to RCC, placing it as the seventh most common cancer in men [9]. In 2022, Kallam and Padala [10] found that RCC is the most common type of urogenital cancer placing it as the sixth most common cancer in men and tenth in women with an extremely high mortality rate for urogenital cancers of 30-40%. Additionally, sixty percent of cases were found to be detected incidentally [10,11]. These studies suggest an increased incidence of RCC in the last four years, which may be due to improved diagnostic procedures such as cross-abdominal screening and increased public awareness on regular screening importance. Concerning age, RCC is most detected in individuals between the ages of 60 and 70 with a declining trend past 70 [11]. Additionally, RCC incidence varies in geographical locations with developed countries such as North America and European regions appearing more often compared to the incidence underdeveloped countries such as South/Central America, Asia, and Africa [12] (Figure 2).
Figure 2: Incidence of renal cell carcinoma in different geographical regions. Adapted with permission from Cancer Research UK [12].
Historically, RCC has proven quite difficult to understand as it was originally considered a single, uniform entity. However, knowledge has evolved tremendously in recent years as RCC has recently become known to encompass a spectrum of various diseases with differing histological subtypes, distinctive genetic and molecular alterations, and consequently various forms of treatment and responses to therapies [9]. RCC is a heterogeneous disease that arises from epithelial cells of the renal cortex or renal tubule. It incorporates clear cell RCC (ccRCC), papillary RCC (pRCC), and chromophobe RCC (chRCC) which account for about 85% of all primary renal cancers with the remaining 15% being transitional cell carcinoma, Wilms tumor, tumors of the collecting ducts, and renal sarcomas. Each of these diseases differs in biology, genetics, and behaviors which explains its historically difficult nature for successful treatment [9,10]. ccRCC’s individual cells tend to appear tremendously pale or clear and is a widely recognized type of RCC, representing about 80% of individuals with kidney malignancy [13]. pRCC cancer cells make finger-like projections called papillae and is the second most basic type of RCC with around 10% of individuals observed to have this shape [13]. chRCC cells are like ccRCC cells with a pale coloring, however, are significantly bigger and stand as the third generally normal type of RCC representing about 5% of cases [14].
Current Treatment Options: Radiotherapy, Chemotherapy, Adjuvant Therapy, and Surgery/Transplantation
Currently, radiotherapy is one of the most accepted modern forms of cancer treatment. There are multiple modes of radiation delivery to the cancer location - external beam radiation and brachytherapy. External beam radiation is delivered from outside the body aiming directly toward the tumor location. Brachytherapy, or internal radiation, is carried out internally by radioactive sources and sealed by catheters or seeds directly into the tumor site. This method is primarily used in treatment of gynecological and prostate malignancies or situations of retreatment [15]. Radiotherapy functions to deprive cancer cells of their multiplication potential. The radiation itself serves as ionizing radiation that forms ions which deposit energy in the targeted tissue and kill cancer cells or cause genetic changes culminating in cancer cell death. However, this specific form of treatment remains difficult to fully understand as tumors differ in their sensitivity to radiation treatment. It can be given with the intent to cure but also function to relieve symptoms and/or combine with other treatment forms [15]. However, in focusing specifically on treatment of RCC, renal cell carcinoma is considered a radioresistant tumor despite radiotherapies ability to decrease the risk of local recurrence after surgery [16,17]. Complications with radiotherapy commonly occur in tissues with slow turnover such as the brain, kidney, liver, wall of intestine, subcutaneous tissue, fatty tissue, and muscle. Consequences in such tissues include fibrosis, atrophy, necrosis, and vascular damage [18]. Radiation therapy in kidneys, specifically, is limited by vascular, glomerular, and tubulointerstitial damage. Ionizing radiation causes double-stranded break in the DNA, followed by apoptosis and necrosis of renal endothelial, tubular, and glomerular cells [19]. Therefore, due to the kidney’s radio-resistant nature, struggles to utilize radiotherapy in RCC treatment remain prevalent in the medical field today.
Another common modern form of cancer treatment is chemotherapy. Chemotherapy utilizes drugs to inhibit cell proliferation and tumor multiplication ideally to prevent invasion and metastasis. Traditional practice of this treatment either affects the appropriate functioning of the preformed molecule or interferes with DNA, RNA, or protein synthesis to influence macromolecular synthesis and function of neoplastic cells. Sufficient interfering of macromolecular synthesis triggers apoptosis, or cell death. However, the process often results in toxic effects because of its effect on normal cells as well [20]. Cytotoxic chemotherapy has been extensively studied in metastatic RCC itself. Most responses are modest, and the mechanisms or resistance remain poorly understood. Targeted therapies have shown some improved outcomes, but most patients relapse and die of their disease [21]. Kidney cancer cells usually do not respond well to chemotherapy drugs except for a few including 5-fluoracil (5-FU), gemcitabine, and vinblastine, which have resulted in beneficial outcomes [22-25]. Overall, though, this treatment form remains not a standard treatment option due to unsatisfactory clinical outcomes. Additionally, there remains a common issue of distinguishing patients who warrant chemotherapy from those who benefit from it [26].
While radiotherapy and chemotherapy individually play a major role in modern cancer treatment, they are often coupled together or with other treatment methods to ensure the most promising outcome. When thinking about the treatment process of cancer and RCC specifically as a whole, adjuvant therapy is often utilized. Adjuvant therapy involves additional cancer treatments, often consisting of chemotherapy, radiotherapy, hormone therapy, targeted therapy, or biological therapy, given after the primary treatment to lower the risk of the disease recurring [27]. When analyzing effective treatment methods of RCC itself, surgical processes such as radical nephrectomy, laparoscopic radical nephrectomy, and nephron-sparing surgery, are currently the standard of care [15]. In localized carcinomas radical nephrectomy remains the standard treatment and provides on average 5-year survival rates up to 98 percent [28]. Radical nephrectomy is the surgical removal of the whole kidney, fatty tissues surrounding the kidney, and ureter, or the portion of the tub connecting the kidney to the bladder [29]. Laparoscopic radical nephrectomy (LRN) is the gold-standard for treatment of clinically localized RCC due to the ability to constitute minimal invasiveness, low death rates, minimal intraoperative blood loss, and fast rehabilitation [30,31]. Nephron-sparing surgery is associated with a higher rate of operative complications - up to 40% - and higher risk of local recurrence [28]. Antonelli et al. performed a study comparing the oncological outcomes of nephron-sparing surgery versus radical nephrectomy in intracapsular RCC’s up to 7cm. They concluded the following results: worse progression and disease-free survival rates for tumor’s equal to 4cm but no significant impact from the type of surgery – whether nephron-sparing or radical[32].Unfortunately, about 1 in 3 people with kidney cancer will have metastasized to the other body parts upon the time of diagnosis. The lungs, lymph nodes, bones, and liver are the most common sites of spread. Surgical removal of metastasis may relieve pain and other symptoms but often does not help people live longer. However, in rare cases, surgery may lead to long-term survival where there is a single metastasis or a few that can be easily removed [33].
When looking at kidney cancer, it is imperative to discuss transplantation. Kidney injuries, such as cancer, provoke a high request of organ transplants making it the most demanding system in the field of regenerative medicine [34].
Organoids
A promising alternative to the conventional cancer models discussed above is based on the discovery of adult stem cells (ASC’s) that proliferate and spontaneously self-organize into three-dimensional organotypic cellular structures, termed organoids, in culture [35]. Organoids were named ‘Method of the Year 2017’ by Nature Methods, which shows promise of this rapidly expanding field that holds high expectation for future therapeutic applications and advances in the medical field [36]. An organoid, by definition, is an in vitro 3D multicellular structure derived exclusively from primary tissue, ESCs or iPSCs, capable of self-renewal and self-organization, and exhibiting similar organ functionality as the tissue of origin [37,38]. Organoids can be established for an increasing variety of organs, including but not limited to gut, stomach, kidney, liver, pancreas, and mammary glands either from tissue-resident adult stem cells (ASCs), direct biopsy samples, pluripotent stem cells (PSCs) such as embryonic stem cells (ESCs), or induced PSCs (iPSCs), obtained from reprogramming differentiated cell types [39]. In standard ASC derived organoid cultures, single stem cells are seeded into a matrix that mimics essential aspects of the niche in which the cell normally reside [38,39]. If proper signaling conditions that target key regulatory pathways are present, the cells undergo proliferation, differentiation, migration, and selection. Despite the absence of spatially organized cues, they form structures that possess the ability to undergo symmetry breaking and acquire an exceptional degree of architectural complexity, even to the extent of mirroring the organization and cell type diversity of their in vivo organ counterpart [39]. PSC-derived organoids are promising platforms for organ modelling and modelling developmental processes of organogenesis of those organs from which tissue samples cannot be easily obtained such as the brain. PSC development depends on the stochastic nature of the differentiation process, which, ultimately leads to high heterogeneity among organoids and proves extremely important in cancer research due to the heterogeneous nature of cancer, specifically RCC [39]. Isolation of human PSCs had expanded the potential to study human biology as never before because of their ability to be derived from the inner cell mass of a human blastocyst or reprogrammed from human somatic cells using a combination of transcription factors. These are referred to as human ESCs and iPSC respectively. PSC scan then be coaxed down into a pathway of differentiation utilizing endogenous developmental cues, in either adherent or aggregate cultures, to study cell types of a particular lineage [40] (Figure 3).
Figure 3: Pluripotent stem-cell derived organoids [36].
Patient-derived tumor organoids have become a favorable model for cancer treatment because, in comparison to traditional 2D culture systems, 3D organoids better resemble the native organ in terms of gene and protein expression, metabolic function, and microscale tissue architecture [38,39] (Figure 4). PDTOs possess self-organizing capabilities and can be cultivated from ESCs or iPSC to mimic embryonic developmental processes, or from ASCs to model tissue homeostasis or disease progression.
Figure 4: Method for Development of Patient-derived Tumor Organoids [41].
Advantages of PDTOs include their ability to expand with high efficiency, cultured for long terms, and eventually cryopreserved. Cryopreservation is a process that preserved organelles, cells, tissues, or any other biological constructs by cooling the samples to very low temperatures [38]. These features improve capabilities of generating biobanks and providing a platform for high-throughput drug screens at lower costs than animal models with fewer ethical concerns [42]. Drug screening on specific patient’s tumors permits personalized therapy predictions as PDTOs can be generated and expanded in vitro in a timeline compatible with therapeutic decision-making [38]. Additionally, the establishment of organoids from healthy tissue of the same patient gives the opportunity to develop less toxic frugs by screening for compounds that selectively kill tumor cells while leaving healthy cells unharmed. Finally, the self-renewing hepatocyte organoid cultures may be used to test for hepatotoxicity – one of the main causes of drug failure in many clinical trials – of a new drug [43] (Figure 5).
Figure 5: Applications of organoid technology for studying development, homeostasis, and disease [37].
Disease Modeling and Drug Therapy Testing
Advances in PDOs during the last two decades has shed light on the precision medicine of RCC as PDOs can serve as a model preserving the majority of the patients’ tumors’ characteristics to predict the drug response of specific individuals [44] (Figure 6). Fatehullah et al. [37] achieved a breakthrough in the field of regenerative medicine with the successful production of mesoderm-derived organoids. Specifically, researchers were able to generate renal organoids by manipulating the GSK3Beta and FGF signaling pathways in human induced pluripotent stem cells (iPSCs) to drive them towards an intermediate mesodermal state. These organoids accurately replicated the segmentation and morphology of fetal human nephrons, including ducts, tubules, and glomeruli. These human renal organoids provide a three-dimensional model to study the development and pathogenesis of renal diseases under controlled conditions, thereby overcoming various limitations of previous models such as two-dimensional monolayers, short-term three-dimensional aggregates, and co-cultures with mouse fibroblasts [37]. (Figure 6).
Figure 6: Graphical Abstract of Patient-derived RCC Organoids for Personalized Cancer Therapy [26].
[45] published a study regarding TKIs role in remaining key targeted therapeutics for first and second line RCC therapy. The experiment consisted of two trials – one for Sunitinib and the other for Pazopanib. The Sunitinib trial consisted of random enrollment of 750 patients with metastatic ccRCC who underwent first line treatment with sunitinib versus interferon-a (IFN-a). The results displayed a PFS (progression free survival) of 11 months for sunitinib compared to 5 months for the IFN-a with an objective response rate of 31% and 6%, respectively. Adverse effects of fatigue, hypertension, diarrhea, and hand-foot syndrome reaction were also reported for both treatments. Historically, sunitinib has been approved by the FDS since 2006 as a front-line monotherapy in patients with advanced RCC. As for the other trial, Pazopanib is another oral multitargeted TKI that gained FA approval in 2009 as a first-line treatment for mRCC. This trial consisted of random enrollment of 435 patients with mRCC of intermediate risk. A PFS increase at 9.2 months but no significant improvement in overall median survival was noted. However, the experiment suggested the lack of significant improvement could be attributed to extensive crossover and use of other treatments upon progression to the Paxopanib arms. Unfortunately, TKI agents do not currently hold the most promising future as most agents gained FDA approval solely by showing improvement in PFS. Additionally, only a few allow for head-to-head comparison which often proves difficult for clinicians to choose the optimal treatment for their patients. Therefore, further investigation is needed to determine the optimal sequence and timing of treatments to be set [45].
[34] conducted a study utilizing normal and tumor RCC organoids that were previously maintained in heterogenous multi-clone stem-like enriched mediums. Researchers began with normal adult kidney specimens from which they were able to isolate and propagate organoids 3D structures composed of both differentiated and undifferentiated cells while retaining nephron specific markers. Previously, normal kidney organoids had only been obtained from PSCs. However, this experiment proved capabilities in the establishment and characterization of normal kidney and ccRCC organoid cultures derived from freshly explanted and frozen patients’ tissues (Figure 7). Normal kidney organoid cultures hold an exceptional potential of applicability in disease modeling, organ replacement therapies, nephrotoxicity tests and developmental studies in addition to providing a normal control for oncological studies. This experiment optimized a new organoid model using ASC-derived organoids that provide a promising option for drug screenings and nephrotoxicity studies as well as reproducing drug responses and metabolism or toxic effects reflective of the patient. Additionally, they found that adjusting the composition of growth factors in the medium may allow for direction of stem cell differentiation towards a specific fate, which, in turn, would permit re-growth of specific segments of nephrons or other selective pieces of the renal organ. Aside from establishing normal kidney organoid cultures, Grassi et al. was able to cultivate organoids derived from cancer tissues although with an inferior success rate to that of their normal counterpart. Their data suggests an innovate approach of a long-term establishment of normal and cancer-derived renal organoids obtained from freshly dissociated adult tissues. The study paves the way to organ replacement pioneering strategies in addition to new models for studying drug-induced nephrotoxicity and renal diseases. Furthermore, they have also opened opportunities for generation of preclinical models aimed at improving therapeutic treatments [34].
Figure 7: Establishment of normal kidney organoid cultures – Section B: top panel [34].
More recently, [41] developed an ex vivo drug testing assay using PDTO cultures. Surgical tumor specimens from 20 patients with RCC were freshly resected and developed ex vivo with the intent to evaluate their histopathological and molecular characteristics using histological staining and whole-exome sequencing (WES). Then, researchers utilized cell viability assay to determine the efficacy of standard TKIs in RCC TOs. Using WES, a strong correspondence was identified at the genetic level between primary tumors and their corresponding TO counterparts. Using PDTOs, a prototype of an ex vivo drug testing assay was successfully developed, and researchers corroborated that RCC TOs exhibited differential responses to TKIs such as sunitinib, pazopanib, cabozantinib, and axitinib treatment which proves consistent with previous studies [45]. PDTOs of RCC cultures possessed similar features of RCC clinical tumors including morphology, genetic alterations, and the expression of the Bcl-2 anti-apoptotic protein. This implies potential use for PDTOs as a model for personalized treatment of RCC patients. Overall, the predictive value of the current assay must be tested and validated in future clinical studies, but the findings demonstrate a novel approach for ex vivo drug testing in PDTO models [41].
Limitation of Organoids
Regarding organoid studies on kidneys, the functional capabilities of the hPSC cultures are often no match to their natural counterparts [36]. Limitations of organoids can be characterized by off-target cells, vascularization, and reproducibility. Kidney organoids derived from established protocols may contain up to 20% of the normal cell population. These off-target cells increase in prevalence as the organoid gets larger and eventually disrupts its integrity. Vascularization also provides a difficult obstacle to overcome as, to date, there is no kidney organoid that shows patent vasculature. Organoids lack sufficient endothelial cells and the proper guiding cues from the vasculature. As organoids grow larger, cell death in the center mass becomes inevitable due to the absence of vascular nutrients and waste exchange. Therefore, the lack of blood vessels and immune cells are an intrinsic limitation of organoid technology [46]. Lastly, current kidney organoids are roughly 1/10,000 of a single human kidney by nephron counts making reproducibility an extremely prevalent limitation in organoid research.
Conclusion
Current treatment options for RCC including radiotherapy, chemotherapy, and surgery have provided few beneficial outcomes for patients because of the kidneys’ high degree of heterogeneity and risk of metastasis. Radiotherapy has often proven successful in depriving cancer cells of their multiplication potential in addition to reducing symptoms and the risk of local recurrence after surgery. However, kidneys are considered a radioresistant tumor and therefore causing complications in most radiotherapy treatments for RCC. As for chemotherapy, few trials have proved successful, and most patients relapse and die of their disease. Surgical treatment is the most common treatment method but has not been deemed overly successful due to the high metastatic risk of RCC. Researchers have found that stem cell and patient-derived tumor organoids, such as PSCs and ASCs, provide a substantially similar, patient-specific, organ model that mirrors its in vivo organ counterpart paving the way for personalized treatment and notable drug-induced nephrotoxicity studies. While the end goal is to cure the disease, the research outlined in this comprehensive study serves as hopeful first steps towards such improvements.
Moving forward, it is imperative to continue research regarding organoids and their benefits in relation to RCC. However, as researchers hopefully come closer and closer to a cure, the availability of obtaining a said successful treatment must be considered. The United States and surrounding world are made up of an astronomically broad degree of socioeconomic statuses. Will this curative treatment have a beneficial or limiting cost and would that cost allow for the treatment to be provided to most people or just the top 5% in gross income. These are questions that are often overlooked but play a major part in the overall efficacy of a curative treatment.
References
- https://www.who.int/news-room/fact-sheets/detail/cancer
- https://seer.cancer.gov/statfacts/html/livibd.html
- https://www.webmd.com/cancer/guide/cancer-incidence-age
- Centers for Disease Control and Prevention. United States Cancer Statistics: Highlights from 2019 Incidence. USCS Data Brief, no. 29. Atlanta, GA: Centers for Disease Control and Prevention, US Department of Health and Human Services; 2022.
- https://www.cancer.gov/about-cancer/understanding/what-is-cancer
- Vogt PK. (1993) Cancer genes. West J M. 158(3): 27378.
- Linehan WM, Ricketts CJ. (2019) The Cancer Genome Atlas of renal cell carcinoma: findings and clinical implications. Nat Rev Urol. 16(9):539–52.
- https://www.cancer.gov/pediatric-adult-rare-tumor/rare-tumors/rare-kidney-tumors/clear-cell-renal-cell-carcinoma#:~:text=Clear%20cell%20renal%20cell%20carcinoma%2C%20or%20ccRCC%2C%20is%20a%20type,called%20conventional%20renal%20cell%20carcinoma.
- Bahadoram S, Davoodi M, Hassanzadeh S, Bahadoram M, Barahman M,et al. (2022) Renal cell carcinoma: an overview of the epidemiology, diagnosis, and treatment. G Ital Nefrol. 39(3).
- Jonasch E, Gao J, Rathmell WK. (2014) Renal cell carcinoma. BMJ. 349:g4797.
- Hsieh JJ, Purdue MP, Signoretti S, Swanton C, Albiges L, et al. (2017) Renal cell carcinoma. Nat Rev Dis Primers. 3(1).
- Chow WH, Dong LM, Devesa SS. (2010) Epidemiology and risk factors for kidney cancer. Nat Rev Urol. 7(5), 245–57.
- Bakitas, MA. (2007) Background Noise: The Experience of Chemotherapy-Induced Peripheral Neuropathy. Nurs Res. 56(5):323-31.
- Singh D. (2021) Current updates and future perspectives on the management of renal cell carcinoma. Life Sci. 264:118632.
- Baskar R, Lee KA, Yeo R, Yeoh KW. (2012) Cancer and Radiation Therapy: Current Advances and Future Directions. Int J Med Sci. 9(3), 193–99.
- De Meerleer G, Khoo V, Escudier B, Joniau S, Bossi A, et al. (2014) Radiotherapy for renal-cell carcinoma. The Lancet Oncol. 15(4):e170–e177.
- Dengina N, Tsimafeyeu I, Mitin T. (2017) Current Role of Radiotherapy for Renal-Cell Carcinoma: Review. Clinical Genitourin Cancer. 15(2):183–87.
- Majeed H, Gupta V. (2022) Adverse Effects Of Radiation Therapy.
- Klaus R, Niyazi M, Lange-Sperandio B. (2021) Radiation-induced kidney toxicity: molecular and cellular pathogenesis. Radiat Oncol. 16(1).
- Amjad MT, Chidharla A, Kasi A. (2023) Cancer Chemotherapy.
- Diamond E, Molina AM, Carbonaro M, Akhtar NH, Giannakakou P, et al. (2015) Cytotoxic chemotherapy in the treatment of advanced renal cell carcinoma in the era of targeted therapy. Crit Rev Oncol Hematol. 96(3):518–26.
- Lilleby W, Fosså SD. (2005) Chemotherapy in metastatic renal cell cancer. World J Urol. 23(3):175‐79.
- Stadler WM, Huo D, George C, Yang X, Ryan CW, et al. (2003) Prognostic factors for survival with gemcitabine plus 5‐fluorouracil based regimens for metastatic renal cancer. J Urol. 170(4 pt 1):1141‐45.
- Buti S, Brighenti M, Bongiovanni C, Buzio C, Chiesa MD, et al. (2007) Role of chemotherapy with gemcitabine plus 5‐fluorouracil and chemoimmunotherapy in metastatic renal cell cancer (mRCC). J Immunother. 30(7):780‐86.
- Roubaud G, Gross‐Goupil M, Wallerand H, de Clermont H, Dilhuydy MS, et al. (2011) Combination of gemcitabine and doxorubicin in rapidly progressive metastatic renal cell carcinoma and/or sarcomatoid renal cell carcinoma. Oncology. 80(3‐4):214‐18.
- Li Z, Xu H, Yu L, Wang J, Meng Q, et al. (2022) Patient‐derived renal cell carcinoma organoids for personalized cancer therapy. Clin Transl Med. 12(7):e970.
- https://www.cancer.gov/publications/dictionaries/cancer-terms/def/adjuvant-therapy
- Staehler G. Brkovic D. (1999) The role of surgery in renal cell carcinoma. Der Urologe, 38(5):452–59.
- https://www.mayoclinic.org/tests-procedures/nephrectomy/about/pac-20385165#:~:text=Radical%20nephrectomy.,or%20involves%20the%20adrenal%20gland
- Asimakopoulos AD, Miano R, Annino F, Micali S, Spera E, et al. (2014) Robotic radical nephrectomy for renal cell carcinoma: a systematic review. BMC Urol. 14:75.
- Naghiyev RN, Imamverdiyev SB, Sanlo Om. (2016) [Laparoscopic radical nephrectomy]. Urologiia. 2-67-70.
- Antonelli A, Cozzoli A, Nicolai M, Zani D, Zanotelli T, et al. (2008) Nephron-Sparing Surgery versus Radical Nephrectomy in the Treatment of Intracapsular Renal Cell Carcinoma up to 7cm. Eur Urol. 53(4):803-9.
- https://www.cancer.org/cancer/kidney-cancer/treating/surgery.html
- Grassi L, Alfonsi R, Francescangeli F, Signore M, De Angelis ML,et al. (2019) Organoids as a new model for improving regenerative medicine and cancer personalized therapy in renal diseases. Cell Death & Disease, 10(3).
- Kretzschmar K. (2020) Cancer research using organoid technology. J Mol Med(Berl). 99(4):501–15.
- Method of the Year 2017: Organoids. (2018). Nat Methods. 15(1):1–1.
- Fatehullah A., Tan, S. H., & Barker, N. (2016). Organoids as an in vitro model of human development and disease. Nature Cell Biology, 18(3), 246–254. https://doi.org/10.1038/ncb3312
- Cerullo, V. (2023). Retrieved April 19, 2023, from Aacrjournals.org website: https://aacrjournals.org/cancerres/article/81/12/3149/666855/Patient-Derived-Organoids-for-Precision-Cancer
- Hofer M, Lutolf MP. (2021) Engineering organoids. Nat Rev Mater. 6(5):402–20.
- Andrews MG, Kriegstein AR. (2022) Challenges of Organoid Research. Annu Rev Neurosci. 45(1):23–39.
- Kazama A, Anraku T, Kuroki H, Shirono Y, Murata M, et al. (2021) Development of patient‑derived tumor organoids and a drug testing model for renal cell carcinoma. Oncol Rep. 46(4):226.
- van de Wetering M, E Francies H, MJoshua F, Bounova G, Iorio F, et al. (2015). Prospective Derivation of a Living Organoid Biobank of Colorectal Cancer Patients. Cell. 161(4):933–45.
- Junttila MR, de Sauvage FJ. (2013) Influence of tumour micro-environment heterogeneity on therapeutic response. Nature. 501(7467):346–54.
- Wang B, Xue Y, Zhai W. (2022) Integration of Tumor Microenvironment in Patient-Derived Organoid Models Help Define Precision Medicine of Renal Cell Carcinoma. Front Immunol. 13:902060.
- Tolia M, Zerdes I, Kyrgias G, kadarnakis D, Tsekeris P, et al. (2019) Systemic therapy of metastatic renal cell carcinoma: Review of the current literature. Urologia. 86(1):3-8.
- Calandrini C, Schutgens F, Oka R, Margaritis T, Candelli T, et al. (2020) An organoid bio bank for childhood kidney cancers that captures disease and tissue heterogeneity. Nat Commun. 11(1):1310.