Comparison of Zygomatic Implants and Implantize Compact from Boneeasy: A Finite Element Analysis for Bending and Safety Factor
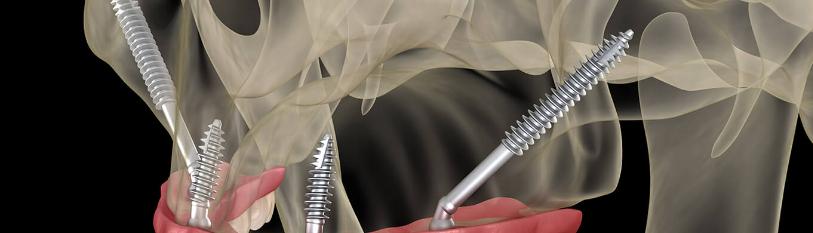
Luís M. M. Santos1, José L. T. Figueiredo2, Rui P. L. M. Coelho3, Sara D. G. Carvalho4 and Maria I. Martins5
1Private practice at Clinica Luís Mesquita Santos, Portugal
2Private practice at Lusíadas Hospital Oporto, Portugal
3Project Manager at Boneeasy research Center, Portugal
4Boneeasy research center
5FEUP, Oporto, Portugal
*Corresponding author: Rui P. L. M. Coelho, Project Manager at Boneeasy research Center, Portugal.
Citation: Santos LMM, Figueiredo JLT, Coelho RPLM, Carvalho SDG, Martins MI. Comparison of Zygomatic Implants and Implantize Compact from Boneeasy: A Finite Element Analysis for Bending and Safety Factor. J Oral Med and Dent Res. 5(3):1-9.
Received: July 22, 2024 | Published: August 02, 2024
Copyright© 2024 genesis pub by Santos LMM, et al. CC BY-NC-ND 4.0 DEED. This is an open-access article distributed under the terms of the Creative Commons Attribution-Non Commercial-No Derivatives 4.0 International License. This allows others distribute, remix, tweak, and build upon the work, even commercially, as long as they credit the authors for the original creation
DOI: https://doi.org/10.52793/JOMDR.2024.5(3)-65
Abstract
The history of maxillofacial implants dates back to ancient civilizations where rudimentary attempts to replace missing facial structures were made using materials like ivory and wood. Significant advancements occurred in the 20th century with the development of biocompatible materials and modern surgical techniques. The introduction of titanium implants by Dr. Per-Ingvar Branemark in the 1960s revolutionized the field due to titanium's excellent osseointegration properties.
Zygomatic implants were developed as a specialized solution for patients with severe maxillary bone loss, where traditional dental implants are not feasible [1-9]. Today, maxillofacial implants are extensively used for trauma reconstruction, congenital defects, and post-oncological surgeries, continually evolving with advancements in material science and 3D printing technologies(c15). This study compares the performance of zygomatic implants and Implantize Compact from Boneeasy through Finite Element Analysis (FEA), focusing on bending and safety factors. The principle underlying this comparison is that for an implant to achieve osseointegration, the micro-vibration should be under 50 microns [1, 2, 10-14, 14-22]. Zygomatic implants were analyzed under various conditions, and their results were compared with those of the Implantize Compact system. Results showed that zygomatic implants exceed the micro-vibration threshold for osseointegration, while the Implantize Compact system remains within acceptable limits, suggesting its higher potential for successful osseointegration.
Keywords
Zygomatic Implants; Implantize Compact from Boneeasy; Maxillofacial implants; Osseointegration
Introduction
Zygomatic implants were pioneered by Dr. Per-Ingvar Brånemark in the late 1980s and early 1990s as an innovative solution for patients with severe maxillary bone loss, where conventional dental implants were not an option. These implants are anchored in the zygomatic bone, or cheekbone, which provides a dense and stable foundation for the prosthetics. The technique initially addressed the needs of cancer patients and those with significant maxillary atrophy. Over the decades, zygomatic implants have evolved with improved designs and surgical techniques, significantly enhancing their success rates and expanding their use in complex maxillofacial reconstructions [1, 2,15-17, 24-27].
Osseointegration is crucial for the success of zygomatic implants, as it ensures a stable and long-lasting foundation for prosthetic rehabilitation. This biological process involves the direct structural and functional connection between the living bone and the surface of the implant, typically made from biocompatible materials like titanium. Effective osseointegration minimizes the risk of implant failure, enhances load distribution, and improves the overall stability and functionality of the implant. For zygomatic implants, which are used in cases of severe maxillary bone loss, achieving robust osseointegration is essential to support the prosthetic structures and to facilitate optimal outcomes in complex maxillofacial reconstructions [17-27].
Finite Element Analysis (FEA) is a crucial computational tool in the field of implant dentistry, particularly for zygomatic implants. It simulates and assesses the mechanical behavior of implants and the surrounding bone, predicting stress distribution and identifying potential failure points at the bone-implant interface. This information is vital for understanding and optimizing osseointegration, the process where the bone forms a direct structural and functional connection with the implant. Optimal stress distribution, revealed through FEA, promotes a favorable biological response, enhancing bone growth and implant stability. By allowing for the refinement of implant designs and surgical techniques, FEA helps ensure robust osseointegration, which is essential for the long-term success and load-bearing capacity of zygomatic implants.
This study aims to compare zygomatic implants and the Implantize Compact system from Boneeasy using Finite Element Analysis (FEA) to determine their potential for osseointegration. According to Brånemark et al. (2001), for successful osseointegration, micro-vibrations should be under 50 microns, highlighting the critical role of mechanical stability in implant success. The study evaluates the minimum safety factor and total displacement of both implant systems to assess their performance under varying loading conditions, aiming to optimize design and surgical techniques for enhanced bone integration and long-term implant durability. By leveraging FEA, this research contributes to advancing implant dentistry by providing insights into stress distribution and biomechanical interactions critical for achieving robust osseointegration in complex maxillofacial reconstructions.
Material and Method
The study involved testing implants of varying lengths (30, 40, 50, and 57.5 mm) at different angulations (30, 40, and 50 degrees) to the occlusal plane. Zygomatic implants engaged the zygomatic bone at an average depth of 7 mm (Figure1,2).
Figure 1: The 7mm constriction of the Zygomatic implant.
Figure 2: Applied force of 200 Nw at 50º.
Implantize Compact system involved a complex configuration with multiple screws for enhanced fixation. An occlusal force of 200 Nw, perpendicular to the occlusal plane, was applied, and FEA was performed using Autodesk Fusion 360 (Figure 3,4).
Figure 3: Constrictions for the Implantize Compact.
Figure 4: Applied force of 200 Nw.
Results
Zygomatic Implants
Figure 5: Amount of displacement and safety factor simulation.
Fixation Length (mm) |
Implant Length (mm) |
Angle (º) |
Minimum Safety Factor |
Total Displacement (mm) |
7 |
30 |
30 |
0.5341 |
0.2452 |
40 |
30 |
0.4213 |
0.3151 |
|
50 |
30 |
0.3493 |
0.3754 |
|
7 |
40 |
30 |
0.5053 |
0.6875 |
40 |
30 |
0.3972 |
0.8837 |
|
50 |
30 |
0.3357 |
1.053 |
|
7 |
50 |
30 |
0.392 |
1.481 |
40 |
30 |
0.3075 |
1.903 |
|
50 |
30 |
0.2594 |
2.268 |
|
7 |
57.5 |
30 |
0.314 |
2.366 |
40 |
30 |
0.246 |
3.042 |
|
50 |
30 |
0.2074 |
3.625 |
Table 1: Zygomatic Implants Results.
Graphic 1: Decrease of the safety factor in function of the increasing of the length and the angle of the force.
Graphic 2: Increase of displacement in function of the increasing of the length and the angle of the force.
All tested configurations exceeded the 50-micron threshold for micro-vibrations, with displacement increasing with both implant length and angulation.
Implantize Compact
Figure 8: Displacement and safety factor of Implantize Compact.
Name |
Minimum |
Maximum |
Safety Factor (Per Body) |
4.562 |
15 |
Stress (von Mises) |
1.176E-04 MPa |
214.834 MPa |
1st Principal |
-72.364 MPa |
158.408 MPa |
3rd Principal |
-283.491 MPa |
50.064 MPa |
Normal XX |
-247.482 MPa |
81.736 MPa |
Normal YY |
-126.88 MPa |
140.111 MPa |
Normal ZZ |
-125.335 MPa |
53.814 MPa |
Shear XY |
-28.438 MPa |
73.178 MPa |
Shear YZ |
-41.366 MPa |
46.826 MPa |
Shear ZX |
-54.23 MPa |
75.541 MPa |
Displacement |
||
Total |
0.00 mm |
0.014 mm |
X |
-0.004 mm |
0.001 mm |
Y |
-8.119E-04 mm |
0.011 mm |
Z |
-3.283E-04 mm |
0.011 mm |
Table 2: Implantize Compact Results.
Im+çantize Compact demonstrated minimal displacement, well within the osseointegration threshold, suggesting a higher potential for successful osseointegration.
Discussion
The FEA results indicate that all zygomatic implants tested exhibit displacement well above the 50-micron threshold required for osseointegration. This displacement increases with both implant length and angulation, reaching a maximum in the 57.5 mm implant at 50 degrees. These findings suggest that zygomatic implants, under the tested conditions, may not achieve stable osseointegration and could lead to bone resorption and other complications due to excessive bending. On the other hand, the Implantize Compact system demonstrated minimal displacement (0.014 mm maximum), well within the osseointegration threshold. This suggests that Implantize Compact has a higher potential for achieving stable osseointegration, making it a more reliable option in clinical practice.
Conclusion
The analysis concludes that zygomatic implants, given their high displacement values, cannot be considered Osseo integrated implants under the tested conditions. This bending could potentially lead to bone resorption, posing a risk for patients. In contrast, the Implantize Compact system shows promising results, with displacement values well within the acceptable range for osseointegration. Further discussions and investigations are warranted to explore the implications of these findings and to improve the design and application of zygomatic implants.
References
-
Kawahara H, Kawahara D, Hayakawa M, Tamai Y, Kuremoto T, et al. (2003) Osseointegration under immediate loading: biomechanical stress–strain and bone formation–resorption. Implant dentistry. 12(1):61-8.
-
Pellegrino G, Grande F, Ferri A, Pisi P, Gandolfi MG, et al. (2020) Three-dimensional radiographic evaluation of the malar bone engagement available for ideal zygomatic implant placement. Methods and Protocols. 3(3):52.
-
Adell R, Lekholm U, Rockler B, Brånemark P.-I. (1981) A 15-year study of osseointegrated implants in the treatment of the edentulous jaw. Int J Oral Surg. 10(6):387-416.
-
Brånemark PI, Hansson BO, Adell R, U Breine, J Lindstrom, et al. (1977) Osseointegrated implants in the treatment of the edentulous jaw. Experience from a 10-year period. Scand J Plast Reconstr Surg Suppl. 16:1-132.
-
Geng JP, Tan KB, Liu GR. (2001) Application of finite element analysis in implant dentistry: A review of the literature. J Prosthet Dent. 85(6):585-98.
-
Misch CE. (2005) Contemporary Implant Dentistry. Elsevier Mosby.
-
Sennerby L, Meredith N. (2008) Implant stability measurements using resonance frequency analysis: biological and biomechanical aspects and clinical implications. Periodontol 2000. 47:51-66.
-
Oosterwyck HV, Duyck J, Sloten VJ, Perre GVD, Puers R, et al. (2008) The influence of bone mechanical properties and implant fixation upon bone loading around oral implants. Clin Oral Implants Res. 19(9):778-86.
-
Aparicio C, Rangert B, Sennerby L. (2003) Immediate/early loading of dental implants: a report from the Sociedad Española de Implantes World Congress consensus meeting in Barcelona, Spain, 2002. Clin Implant Dent Relat Res. 5(1):57-60.
-
Roberts WE, Turley PK, Brezniak N, Fielder PL. (1987) Bone physiology and metabolism. J Clin Orthodont. 21(9):604-12.
-
Frost HM. (1994) Wolff's Law and bone's structural adaptations to mechanical usage: an overview for clinicians. Angle Orthod. 64(3):175-88.
-
Steigenga JT, Al-Shammari KF, Misch CE, Nociti FH, Wang HL, et al. (2003) Dental implant design and its relationship to long-term implant success. Implant Dent.12(4):306-17.
-
Manzon L, Arcuri C, Cascone P, et al. (2014). Biomechanical evaluation of angled abutments for oral implants: an in vitro study. Journal of Craniofacial Surgery, 25(3), 815-819.
-
Frost HM. (1964) The Laws of Bone Structure. Charles C. Thomas.
-
Lee JH, Frias V, Lee KW, Wright RF. (2005) Effect of implant size and shape on implant success rates: a literature review. J Prosthet Dent. 94(4): 377-81.
-
Esposito M, Hirsch JM, Lekholm U, Thomsen P. (1998) Biological factors contributing to failures of osseointegrated oral implants. (II). Etiopathogenesis. Eur J Oral Sci. 106(3):721-64.
-
Frost HM. (1997) On our age-related bone loss: insights from a new paradigm. J Bone Miner Res.12(10):1539-46.
-
Klein MO, Schiegnitz E, Al-Nawas B. (2014) Systematic review on success of narrow-diameter dental implants. Int J Oral Maxillofac Implants. 29(Suppl):43-54.
-
Moraschini V, Poubel LADC, Ferreira VF, Barboza EDSP. (2015) Evaluation of survival and success rates of dental implants reported in longitudinal studies with a follow-up period of at least 10 years: a systematic review. International Journal of Oral & Maxillofacial Surgery, 44(3):377-88.
-
Esposito M, Grusovin MG, Coulthard P, Worthington HV, (2007) The efficacy of various bone augmentation procedures for dental implants: a Cochrane systematic review of randomized controlled clinical trials. Int J Oral Maxillofac Implants. 21(4):696-710.
-
Lindhe J, Meyle J. (2008) Peri-implant diseases: Consensus Report of the Sixth European Workshop on Periodontology. J Clin Periodontol. 35(Suppl 8):282-85.
-
Sakka S, Coulthard P. (2009) Bone quality: a reality for the process of osseointegration. Implant Dent.18(6):480-85.
-
Akca K, Cehreli MC. (2006) Biomechanical consequences of progressive marginal bone loss around oral implants: a finite element stress analysis. Med Biol Eng Comput. 44(6):527-35.
-
O'Mahony A, MacCarthy D, Spencer P. (2000) An examination of the bone remodeling surrounding a dental implant fixture subjected to continuous occlusal loading: an analysis using ABAQUS. J Bio Materials Res. 52(4):642-9.
-
Hämmerle, CH, Wagner D, Brägger U. (1995). Threshold of micromotion for bone ingrowth into titanium chambers implanted in rabbit tibiae. Clin Oral Implants Res. 6(3):207-13.
-
Kawahara H, Kawahara D, Hayakawa M, Tamai Y, Kuremoto T, et al. (2003) Osseointegration under immediate loading: biomechanical stress-strain and bone formation--resorption. Implant Dent.12(1):61-8.
-
Pellegrino G, Grande F, Ferri A, Pisi P, Gandolfi MG. et al. (2020) Three-Dimensional Radiographic Evaluation of the Malar Bone Engagement Available for Ideal Zygomatic Implant Placement. Methods Protoc. 3(3):52.