Advances in Mesenchymal Cell Therapy in Dermatology and Aesthetic Medicine
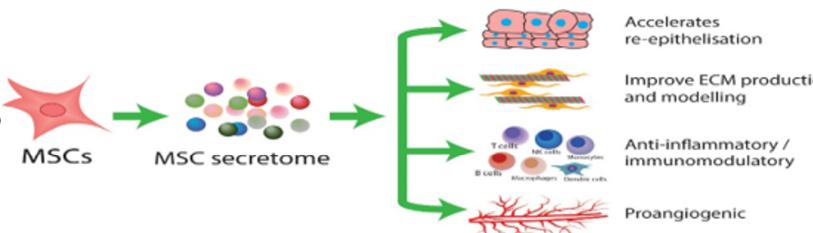
Glen Alvin1 and Mike Chan1,2,3*
1European Wellness Academy GmbH – Germany
2European Wellness Centers, Switzerland
3FCTI Research & Development GmbH – Germany
*Corresponding author: Mike Chan, FCTI Research & Development GmbH – Germany.
Citation: Alvin G, Chan M. Advances in Mesenchymal Cell Therapy in Dermatology and Aesthetic Medicine. J Stem Cell Res. 5(2):1-22.
Received: May 14, 2024 | Published: March 27, 2024
Copyright© 2024 genesis pub by Alvin G, et al. CC BY-NC-ND 4.0 DEED. This is an open-access article distributedunder the terms of the Creative Commons Attribution-NonCommercial-No Derivatives 4.0 International License.,This allows others distribute, remix, tweak, and build upon the work, even commercially, as long as they credit the authors for the original creation
DOI: https://doi.org/10.52793/JSCR.2024.5(2)-S2(1)
Abstract
Stem cells are a group of undifferentiated cells capable of increasing to enkindle differentiated cells. Mesenchymal stem cells are versatile multilineage stromal-derived non-hematopoietic progenitor cells that play an essential role in regenerative medicine. They have the aptitude to execute tissue regeneration by triggering inflammation, angiogenesis, and recruiting tissue-specific progenitors. Mesenchymal stem cells possess excellent qualities like stemness potency, ease of isolation, low risk of malignancy, and therapeutic versatility. The predilection of skin mesenchymal stem cells includes hair follicle stem cells, sebaceous gland stem cells, melanocyte stem cells, interfollicular epidermis stem cells, and dermal stem cells. Exosomes are nano-sized membranous extracellular lipidic vesicles. Exosomes deliver cell-free therapeutics. The task of mesenchymal stem cells in dermatologic therapeutics is establishing order in function and integrity. They refine the skin and appearance and restore previous structural foundations in aesthetics.
Keywords
Mesenchymal stem cells; Dermatology; Aesthetic medicine
Introduction
Stem cells are a population of undifferentiated or partially differentiated cells capable of dividing and proliferating to create differentiated cells [15]. Stem cells span from pluripotent cells unsheathed from blastocysts to progenitor cells from fetal and adult tissues and induced pluripotent stem cells (iPSC) [15]. Tissue stem cells are the foundation for adult tissue regeneration, and their cellular states during homeostasis are tightly regulated by [14].
(i) Intrinsic mechanisms, such as chromatin structure, transcriptional control, and metabolism [14].
(ii) Extrinsic mechanisms encompass the bi‐directional molecular cross-talk between stem cells and their functional niche cells [14].
Organogenesis in mammals is an astounding process [9]. The cells of the 3 germ layers transform into an embryo that later matures to configure major internal and external organs within a short window [9]. The embryonic mesenchymal cell synthesis is one of the most critical events during embryogenesis, leading to the establishment of the major organs and soft tissues [8]. Connective tissues, including bone, cartilage, adipose, blood cells and vasculature, are derived from mesenchymal cells during embryonic growth [8]. The earliest mesenchymal cells, which give rise to primitive streaks, arise from the epiblast through epithelial-to-mesenchymal transition during gastrulation [8]. Following differentiation and migration, these primitive mesenchymal cells partition from the mesoderm into 3 significant components, forming the lateral plate, intermediate and paraxial mesoderm [8]. Another portion of mesenchyme is produced from the ectoderm at the surface and the margins of the neural tube [8, 18].
Mesenchymal stem cells (MSCs) are versatile multilineage stem cells that self-renew, differentiate and proliferate into various cell types that play an essential role in regenerative medicine [1, 2]. They were first observed in bone marrow in 1867 by Cohnheim, who deduced that these cells could be a source of fibroblasts to fuel wound repair [12]. The term "mesenchymal stem cells" was later coined by Caplan in 1991, mooting his studies with human bone marrow [12]. Mesenchymal stem cells (MSCs) are stromal-derived non-hematopoietic progenitor cells in neonatal and adult tissues [29]. MSCs have the aptitude to differentiate cells from skin, bone and cartilage and regenerate their corresponding tissues directly [8]. Mesenchymal tissues are exigent components of any organ in the human body [8]. The mesenchymal tissues of various organs are composed of individual mesenchymal cells that share a common phenotype and potential to grow despite arising from functionally and developmentally diverse cell populations [8]. MSCs can also regenerate tissues indirectly by inflammation, stimulating angiogenesis, and recruiting tissue-specific progenitors to the injury site [8]. They manifest potent immunosuppressive activities, as observed following transplantation [8]. Some of the MSC's qualities that make them a plausible candidate for cell therapy are their regenerative potential, multipotent differentiation and ability to regulate immunological and inflammatory responses [10]. MSCs are characterised by the expression of cell surface markers, including CD44+, CD3+, CD90+, and CD105+, and being negative for markers including HLA‐ DR, CD45, CD14, and CD3416. MSC treatments for various conditions have been pursued in numerous clinical trials, mainly because of their ability to regenerate tissue and organs [8].
These cells represent one of the most widely distributed cells in the ectoderm, forming the epidermal epithelium and body [4]. They are derived from biological sources, including bone marrow, umbilical cord, adipose tissue, kidney, brain, spleen, and liver and neonatal tissues such as umbilical cord and placenta [2 ,7]. A cell must be able to proliferate for self-renewal and cell expansion purposes related to its "stemness" [7]. MSCs refer to a marginal cell population in all organs containing a perivascular niche owing to the expression of stromal cell surface marker-1 (Stro-1) and α-smooth muscle actin (α-SMA), regardless of their source [7]. MSCs have a unique homing ability to migrate to a targeted site [7]. They differentiate and stimulate the secretion of chemokines, cytokines, and growth factors at the targeted region, aiding tissue regeneration [1]. The MSCs differentiate into diverse cells, such as adipocytes, chondroblasts and osteoblasts, under a definite stimulation; this differentiation process is perceived morphologically and with specific biomarkers [7]. They also possess trophic and immunosuppression functions [7]. The International Society of Cell Therapy (ISCT) criteria to define MSCs include [7]:
(1) MSCs must be adherent cells showing a spindle-shape morphology in standard culture conditions [7]
(2) MSCs must show cell surface expression of cluster of differentiation (CD)73, CD90 and CD105, but not CD45, CD34, CD14 or CD11b, CD79α or CD19 and HLA-DR antigens [7].
(3) MSCs must differentiate into osteoblasts, adipocytes and chondroblasts in vitro following a definite stimulation7. This criterion requires an update to remain relevant [7].
Human MSCs generally express markers including CD90, CD73 and CD105; however, these surface marker profiles differ slightly in various tissues [4]. The MSCs from bone marrow are positive for CD73, CD90, CD10and 5, STRO-1 but negative for CD14, CD34, CD45 and HLA-DR, while adipose tissue-derived MSCs are positive for extra CD29, CD44, CD71, CD13, CD166, but negative for CD3 [4].
Once the adoptive transfer has been adjudicated, the MSCs may move along blood vessels, passing through the endothelial wall, until they reach their destined location [7]. This journey is aided by the expression and functionality of adhesion molecules, chemokine receptors, and enzymes belonging to the molecular class of metalloproteinases (MMPs), which are indispensable for enabling the trafficking of MSCs towards specific target organs [7]. The adhesion of MSCs onto microvasculature is dependent on the CD62P receptor expressed on endothelial cells (ECs) and also by integrins, including very late antigen-4 (VLA-4), which interacts with its receptor vascular cell adhesion molecule-1 (VCAM-1) [7]. The CD62P and VCAM-1 expressed on ECs are necessary for MSCs to undergo rolling/adhesion processes [7].
The chemokine receptors expressed variably on the cell surfaces of MSCs include CCR-2,-3,-4- 7,-10, and CXCR-4,-5, and -6 [7]. CXCR-4 is vital to synchronise MSC homing/migration [7]. The activity of the proteolytic enzymes MMPs, particularly MMP-2, enables diapedeses and the interstitial migration of MSCs toward the earmarked tissues [7]. The other MMPs, such as MMP-1, -3 and -9, are also positively associated with MSC homing/migration [7]. The immunomodulatory properties of MSCs succour its migration to inflammatory and tumour sites [4]. MSCs suppress a wide range of immune cells, including T, B, and natural killer (NK) lymphocytes, and influence the functions of myeloid cells such as monocytes, dendritic cells (DCs) and macrophages [7]. They attune the innate and adaptive immune system by disrupting its activation, proliferation, maturation, cytokine production, cytolytic activity, or antibody production [7]. MSCs specifically hamper the effector T-lymphocyte functions such as T helper 17 (Th17) cytokine production while favouring tolerogenic CD4+ Th2 lymphocyte differentiation at the expense of immunity mediated by CD4+ Th1 lymphocytes [7]. They also hinder [7]:
1. B lymphocytes from further differentiating to plasma cells, hence impeding the secretion of immunoglobulins [7]
2. NK lymphocyte's cytotoxic potential and their ability to secrete INF-γ [7]
3. CD14+ monocytes and CD34+ progenitor differentiation to mature DCs [7]
MSCs aggrandise the emergence and recruitment of specific regulatory/suppressive immune subsets, including CD4+CD25+FOXP3+ T lymphocytes, CD8+CD28− T lymphocytes, interleukin-10 (IL-10) producing B lymphocytes, IL-10-producing DCs, and alternatively activated M2-macrophages [7]. This capability amplifies their immunosuppression effects by reinforcing the host's regulatory/immunosuppressive immune subsets [7]. The MSC immunosuppression faculty is mainly executed by generating factors and their paracrine actions on immune cells [7]. MSCs give rise to and release soluble factors accountable for their immunosuppression function, including IL-6, leukaemia inhibitory factor, IL-10, TGF-β, and TNF stimulated gene 6 (TSG-6) and metabolic enzymes, including heme oxygenase-1 (HO-1), indoleamine 2,3 dioxygenase (IDO), and inducible nitric oxide synthase (iNOS) as well as pleiotropic hormones such as prostaglandin E2 (PGE2) and other proteins such as galectin-1, non-classical HLA-class Ib HLA-G, and semaphorin-3A [7].
The immunomodulation footprint of MSC has been extensively explored [4]. MSCs govern lymphocyte proliferation, including the expansion of B cells and their derivates, inducing the embellishment of regulatory T cells, inhibiting natural killer (NK) cell activation, and arresting dendritic cell (DC) functions [4]. The immunomodulatory potentials of MSCs are exerted either by direct cell-to-cell contact or paracrine secretion [4]. The upregulation of cell adhesion molecules like CD274 and galectin-1 is an excellent example of how MSCs deploy their immunomodulatory effects [4]. MSCs also churn out many soluble factors, such as enzymes, cytokines, and nitric oxide (NO), to conciliate immune responses [4] (Figure 1).
Figure 1: Schematic diagram of the Mesenchymal stem cell's secretome mechanism.
The rationale behind why MSCs are ideal for cell therapy is [12]:
-
Stemness potency [12]
-
Easy to isolate [12]
-
Low risk of malignancy [12]
-
Therapeutically versatile [12]
The ability to go home to the site of inflammation trims the immune system response, thus easing tissue regeneration [3].
Exosomes are nano-sized (30–200 nm) membranous extracellular lipidic vesicles (EVs) derived from endosome and plasma membranes through endocytosis, fusion and budding processes mainly employed in cell-free therapeutics [2, 11, 13]. Exosomes convey vital information and macromolecules from their source of origin via intercellular communication and signal transducers [2]. These macromolecules comprise proteins, enzymes, transcription factors, lipids, extracellular matrix proteins, receptors, and nucleic acids [2]. Exosomes are secreted by most cells, including immune cells, such as B cells, T cells, dendritic cells, mast cells, epithelial cells, endothelial cells, neuronal cells, embryonic cells, cancer cells, and MSCs [2].
Proclaimed as the long-sought-after fountain of youth, stem cells, especially MSCs, are the bastion for anti-ageing and other therapies analogous to tissue regeneration [8]. MSCs have been tested as a cellular pharmaceutical in humans since 1995 and have become the most clinically studied experimental cell therapy platform worldwide [5] (Figure 2).
Figure 2: Schematic diagram of the regenerative abilities of exosomes on the skin.
Cutaneous Cell
The skin plays an effectual task in protecting the body against external insults of various natures [22]. It renders a formidable barrier against UV radiation, temperature, exposure to xenobiotics, pollutants, and trauma [22]. The skin must be revamped throughout life, and its adnexal structures, like hair follicles, go through growth and regression in a defined cycle to stay relevant [22]. The skin comprises 3 primary layers: epidermis, dermis, and hypodermis [12]. These layers have distinct structures and function concurrently to protect the internal organs and serve diverse biological processes [12] (Figure 3).
Figure 3: An illustration of the human skin Structure.
There has been immense interest in discerning the regulations and coordination of stem cells within the skin [15]. The predilection of stem cells dispersed on the skin are the hair follicle stem cells (HFSCs), sebaceous gland stem cells, melanocyte stem cells, interfollicular epidermis stem cells, and dermal stem cells [19]. The follicular, epidermal, and melanocyte stem cells lie in the epidermis, while the rest are in the dermis [22]. Regardless of their diverse niche, these stem cells are collectively called skin stem cells [19]. Any form of distortion to the skin stem cell population manifests in a pathological consequence, e.g. a mutation in stem cell genetic material represents the starting point of a possible skin malignancy [22]. Stem cells exhibit versatility; when HFSCs re-epithelialise on the epidermis, they resolve lineage infidelity and adopt the identity of the stem cells in their new niche [21]. HFSCs are particularly useful because of their differentiation ability and self-renewal properties [24]. Irrespective of their frequent battering, Stem cells endure the increasingly strained demands to maintain tissue integrity over a lifetime [21] (Figure 4).
Figure 4: An illustration of the general structure of the skin showing the predilection of skin stem cells.
Stem cell in dermatology
Please see figure 5.
Figure 5: Schematic diagram showing mesenchymal stem cell therapy strategies.
Chronic Wounds
Wound healing is a dynamic, complex biological process in skin tissue after an injury by trauma, burn, or diseases such as diabetes [11]. Cutaneous wounds can be divided into acute or chronic wounds, where chronic wounds are defined as wounds that did not heal within 3 months, alternatively considered non-healing wounds [13]. The Wound Healing Society segregated chronic wounds into 4 sections: pressure ulcers, diabetic ulcers, venous ulcers, and arterial insufficiency ulcers [13]. Injuries to the skin heal by an intricate uninterrupted process that can be broken down based on biological processes into 4 phases [2]:
Hemostasis
The hemostasis phase kicks off with platelet aggregation to form clots to prevent blood loss [2]. These platelets secrete hormones, cytokines, and chemokines, including tumour growth factor-β (TGF-β), platelet-derived growth factor (PDGF), fibroblast growth factor (FGF), and epidermal growth factor (EGF), to lure inflammatory cells [2].
Inflammation
The inflammation phase commences as neutrophils infiltrate the wound and release toll-like receptors (TLRs) and nuclear factor к-light-chain-enhancer of activated B cells (NF-кB) to attract and initiate pro-inflammatory (M1) macrophages [2]. M1 macrophages phagocytose pathogens, remove apoptotic cells, and switch on signal transducer and activator of transcription 3 (STAT-3), which boosts the polarisation of M1 macrophages into anti-inflammatory M2 macrophages, thus stimulating inflammatory resolution [2].
Proliferation
Vascular endothelial growth factors (VEGF) and FGF stimulate angiogenesis, transporting essential nutrients, oxygen, and other growth factors to the damaged tissues [2]. During the proliferation phase, keratinocytes and fibroblasts proliferate at the edge of the wound [2]. Fibroblasts secrete immature type III collagen to create an extracellular matrix (ECM) that eventually gives rise to myofibroblasts [2]. Myofibroblasts are specialised cells with contractile abilities to shrink the wound [2].
Maturation/Remodeling
In the maturation phase, the ECM is degraded by enzymes, including matrix metalloproteinases (MMPs) and plasminogen activators, and type III collagen is substituted by mature to type I collagen [2] (Figure 6).
Figure 6: An illustration of the natural wound healing phases.
The thorny issue in chronic wounds is that non-healing injuries exhibit a prolonged inflammatory phase [26]. The chronic inflammatory state lingers when pro-inflammatory cytokines such as IL-1β, IL-6 and tumour necrosis factor-alpha (TNF-α) are incessantly released into the wound [26]. The resolution of inflammation transpires satisfactorily once the M1 macrophage switches s to M2 polarisation [26].
Non-healing wounds comprise fibroblasts that are not sensitive to TGF-β arousal; hence, they cease to migrate to the wound site, decreasing collagen synthesis, and the outcome is a dysregulated ECM [26].
Conventional wound care methods like skin grafting, skin flap transplantation, laser therapy and biological stents risk atrophic scar formation and pigmentary abnormalities [11]. Alternative treatments include local growth factor administration and gene therapy11. Stem cells promote wound healing in a paracrine way by [11]:
(1) Regulating macrophages, T cells, and B cells (Anti-inflammation) [11]
(2) Secretion of growth factors like VEGF (Angiogenesis)11
(3) Promoting fibroblasts and keratinocyte‐forming cells (Remodelling) [11]
(4) Producing anti‐fibrosis cytokines [11]
(5) Synthesis of endothelial cells and keratinocytes [11]
Stem cells seed scarring mitigation and wound closure acceleration to increase wound healing potential [27]. This is feasible due to their characteristics like pluripotency, self‐renewal and the ability to secrete regenerative cytokines [11].
Cytokines are the main ammunition of MSCs in wound healing [27]. MSCs temper the inflammation phase during wound healing to initiate tissue reconstitution [6]. They exert their role in wound healing in 2 ways [27]:
(A) Stimulation of cellular genesis (fibroblasts, epithelium, keratinocytes) [27]
(B) Paracrine modulation (angiogenesis, neovascularisation, re-epithelisation) [27].
MSCs and dermal fibroblast (DF) migrate to the injured site to regenerate senescent cells and repopulate the skin [6]. Stromal cell‐derived factor-1 (SDF-1) is the constitutive and main protein related to human skin cell migration in damaged tissues [6]. Microenvironment changes induced by the overexpression of SDF-1 in DF lead to keratinocyte proliferation, and with the aid of neovascularisation and increased ECM secretion, the remodelling phase of wound healing flourishes [6]. MSCs aid wound closure by accelerating DF and keratinocyte migration [17]. When cultured in EGF with a high Ca2+ level, MSCs differentiated into keratinocyte-like cells and presented keratinocyte markers such as involucrin, filaggrin, cytokeratin-5, and cytokeratin-10, respectively and stratifin; moreover, they acquired the facility to form stratified structures, as observed in normal skin [6].
Smith et al. attested that MSCs secrete soluble factors such as growth factors, cytokines, chemokines, and hormones that prompt DF proliferation, migration, and chemotaxis [17]. Some growth factors secreted by MSCs include VEGF, PDGF, IGF, HGF, β -FGF, SDF-1, TGF-β, and GDF11 [6], [17]. A clinical trial on non-healing lower limb ulcers using topically applied MSCs demonstrated that the MSC therapy reduced ulcer size and significantly improved pain-free walking distance [17]. Biomarkers indicated that the MSCs improved wound healing through increasing re-epithelialisation and the thickness of the regenerated epidermis [17]. A case report described the injection of human bone marrow MSCs (BM-MSCs) around and within the chronic radiation-induced skin lesions of a 66-year-old patient [31]. The BM-MSCs reduced inflammation, including a marked decrease of β1 integrin expression on lymphocytes and improved skin vasculature and quality [31]. After 3 months, the wound size completely reduced without non-adverse events [31]. Confirmed that autologous MSCs administered to wounds using a fibrin spray system could heal cutaneous wounds [31].
In diabetic patients, high glucose levels thwart the conventional macrophage polarisation from M1 → M2, making these patients prone to chronic skin wounds [2]. A study on 8 non-healing diabetic ulcers treated with a topical combination of MSC, platelets, fibrin glue, and collagen matrix presented successful healing for 3 patients and a significant wound size reduction in the remaining 5 patients [17]. Another study utilising grafts composed of autologous MSCs derived from bone marrow and skin fibroblasts delivered on biodegradable collagen membranes successfully treated patients with diabetic foot ulcers (DFU) [35]. Demonstrated that embryonic MSCs enhance angiogenesis and cell proliferation by secreting growth factors [12]. Embryonic MSCs increase wound closure, vessel formation and the expression of collagen type I and III, fibronectin, and fibroblast-specific protein-1 (FSP-1) [12]. Published evidence that embryonic MSCs promote wound healing in pressure ulcers [12].
The potential of MSC-Exosomes (MSC-Exos), particularly the growth factor and microRNA, to treat chronic skin ulcers and hypertrophic scars has been in the spotlight recently [2] (Figure 7). MSC-Exos mediates wound healing similarly to the principles applied by MSCs [11]:
Figure 7: Schematic diagram showing the mechanism of MSC‐EXOs promoting wound healing.
MSC-Exos earmarks several pathways, including phosphoinositide 3-kinase (PI3K)/AKT, ERK, and STAT-3, which facilitates and accelerates wound healing through downstream targets such as hepatocyte growth factor (HGF), insulin-like growth factor-1 (IGF-1), nerve growth factor (NGF), and stromal cell-derived factor [2]. High blood glucose levels instigate reactive oxygen species (ROS) activity and inflammation, which promotes senescence and impairs the function of EPCs [13]. MSC-Exos accelerate macrophage polarisation via microRNA, as miR-132, miR-126, and miR-21 are highly upregulated during inflammation and attenuate cytokine secretion to resolve the inflammatory stage [2]. and can induce M2 polarisation by regulating TLRs [2]. The microRNA miR-21 impacts several diseases and injuries by promoting the migration of keratinocytes and fibroblasts, stimulating re-epithelialisation and encouraging collagen synthesis [2]. Additionally, miR-21 downregulates TLR-4-mediated inflammation by inhibiting the expression of programmed cell death protein-4 (PDCD4) [2]. MSC-Exos lessens high blood glucose-induced premature senescence of endothelial progenitor cells (EPCs), thus enhancing wound healing in diabetics [13].
Burns are a common injury worldwide12. Burn injuries are classified as first to third-degree burns (1~3°) [12]. Third-degree (3°) burns damage the full thickness of the skin [12]. Their recovery depends on the severity, depth and extent of the burn wounds, and an average of 2 weeks is usually needed for recovery from superficial burn wounds [12]. Conventional treatments for burns are washing the wound daily, removing dead tissues, antibiotic bandaging till the formation of granulation tissue, and transplantation [27].MSCs have proven beneficial therapeutic effects on burn wound healing [17]. A study evaluating a cell spray application of MSCs over third-degree burns versus placebo demonstrated that after 21 days of follow-up, the wounds showed higher re-epithelialisation compared to the control group [31]. The bleeding had ceased entirely by the second-week post-application [31]. Jeschkereported applying allogeneic MSCs topically on a patient with severe burn injuries [31]. The findings revealed 97% of wounds recovered completely with minimal hyperpigmentation or hypertrophic scarring [31]. Studied the effects of hair follicles containing dermal grafts harvested from the scalp to treat patients with third-degree burns [29]. Study results showed that the MSCs in the dermal graft enhanced the re-epithelialisation of burn wounds, comparable to split-thickness skin grafts (STSGs) [29]. The clinical evaluation exhibited that the quality of the new epidermis was good, and histology demonstrated that the thickness of the epidermis was normal [29]. A case report on treating radiation burns by combining surgery and autologous-treated MSCs showed that MSCs successfully modulated radiation inflammatory processes [17].
Atopic Dermatitis
Atopic dermatitis (AD) is a chronic relapsing inflammatory skin disorder affecting up to 20% of children and 3% of adults worldwide [13]. It is characterised by pruritic eczematous lesions, impaired cutaneous barrier function, TH2-type immune system hyperactivation, and elevated serum immunoglobulin E levels [29]. The pathogenesis of AD is multifactorial, with genetic factors, epidermal barrier defects and immunopathogenic factors, where the abnormal activation of T helper 2 (Th2) lymphocyte triggers the secretion of pro-inflammatory cytokines, including immunoglobulin E (IgE), interleukin-4 (IL-4), IL-5, IL-13, IL-17, IL-22, IL-31, and thymic stromal lymphopoietin (TSLP), causing barrier defects and inflammation [28, 29]. The current therapeutic methods vary from topicals to phototherapy to immunosuppressant drugs and biological agents [29]. The underlying mechanism behind the current treatment modalities is inhibiting, not regulating, the abnormal inflammatory response to suppress disease symptoms [28]. Interestingly, the MSCs population of patients with inflammatory skin diseases exhibits an impaired biological capability to influence cellular inflammation, differentiation, and regeneration compared to the healthy population [28]. Point out that MSCs isolated from the skin lesion of patients in AD can enhance the activation of Th1 and Th17 cells and promote the production of pro-inflammatory cytokines, including IL-6, IL-13, IL-17A, IL-17F, transforming growth factor-beta (TGF-β), and IFN-γ; however, there is a decrease in the number of Th2 cells and their production, including IL-2, IL-4, IL-5, and IL-23A [28].
The clinical manifestations of AD differ with age, where adults manifest eczema at the flexural folds, the face, the upper arms and back, hands, feet, fingers and toes. At the same time, infants typically present erythematous papules and vesicles on the cheeks, forehead, neck and scalp. At the same time, children exhibit dry skin, lichenified papules, and plaques in flexural areas of the limbs [29].
MSCs hold tissue repair potential through self-renewal and differentiation calibre [29]. They are potent immune system modulators that amplify immune cells' proliferation, recruitment, and function from innate and adaptive immune systems [29]. The therapeutic effects of MSC include suppression of mast cell degranulation by increasing PGE2 and TGF-β1, rebalancing Th1, Th17, and Th2, and decreasing cytokines or chemokines like IL-1β, IL4, IL5, IL6, IL13, IL17, CCL5, TNF-α, IFN-γ, TSLP and IgE [30]. Intravenously administered allogeneic BM-MSCs to 5 patients with AD 3 times every fortnightly for a month and observed them for a further 12 weeks [28]. They found that the Eczema Area and Severity Index (EASI) improved significantly at week 16, and the therapy had efficacy for an average of 38 weeks post-therapy with no serious adverse effects reported [28]. Pro-inflammatory cytokines significantly decreased by the end of the study [28]. Similarly, Kim et. recruited 34 patients with moderate-to-severe AD who were subcutaneously administered MSCs [28]. After 3 months of therapy, the authors discovered that the treatment effectively reduced symptoms of AD with little adverse effects and no relapse [28] (Figure 8).
Figure 8: Schematic diagram showing the immunologic cascade of atopic dermatitis.
MSC-Exos ameliorates AD by reducing pathological symptoms and expression of multiple cytokines such as IL-4, IL-5, IL-13, IL-17, IL-23, IL-31, TNF-α, IFN-γ, and thymic stromal lymphopoietin (TSLP) [13]. Th2 cytokines, such as IL-4, IL-5, IL-13, and IL-31, produced by activated Th2 cells are crucial contributing factors for inciting cutaneous allergic reactions13. Th2 cytokines, including IL-4, IL-13, and IL-31, are therapeutic targets in AD treatment [13]. MSC-Exos also depleted the infiltration of inflammatory dendritic epidermal cells (IDECs, CD86+, and CD206+), releasing pro-inflammatory cytokines at lesional skin [13]. MSC-Exos exalt skin regeneration by promoting macrophage M2 polarisation with anti-inflammatory properties and diminishing pro-inflammatory cytokine-releasing cells such as M1 macrophages and inflammatory dendritic epidermal cells (IDECs) [13]. MSC-Exos induced cutaneous barrier restoration by yielding ceramides and dihydroceramide in long acyl chains in a dose-dependent manner [13]. MSC-Exos promote sphingoid synthesis, including sphingosine and S1P, increases SphK1 activity, and reduces S1P lyase (S1P1) activity in the injured skin [13].
The S1P/Sphk1/S1PR axis is essential for inducing M2 macrophage polarisation by MSC-Exos, which reduces inflammation and promotes cutaneous wound healing [13]. MSC-Exos also increases the quantity of epidermal lamellar bodies and the lamellar layer's formation at the stratum corneum's interface and stratum granulosum [13]. Transcriptome analysis of diseased skins revealed that MSC-Exos reverses the abnormal expression of genes involved in skin barrier maintenance, lipid metabolism, the cell cycle, and inflammatory responses induced by repeated oxazolone exposures [13]. These results suggest that MSC-Exos could be a promising cell-free treatment for regenerating the skin barrier in various diseases with skin barrier defects [13]. Investigated the safety and efficacy of human mesenchymal stem cell-derived exosomes (MSC-exosomes) applied topically twice daily on 22 adult females diagnosed with sensitive skin over 1 month [41]. The results divulged objective symptoms, including roughness, scales, erythema, and subjective symptoms, including tension, burning, or itching, were improved in the subjects [41].
Epidermolysis Bullosa
Epidermolysis Bullosa (EB) is a bullous skin disease characterised by mechanical fragility of the skin and mucosa due to a genetic mutation in the genes associated with the binding of basal epidermal cells and basement membrane proteins [18, 38]. EB can be categorised into 4 classical subtypes, EB simplex, junctional EB, dystrophic EB and Kindler EB, based on prominent clinical features, like blister formation in the skin and molecular pathology [39]. The different EB forms result from recessive or dominant gene mutations encoding structural proteins at or close to the dermal-epidermal junction (DEJ) [39]. EB is associated with chronic inflammation and contributes to disease progression, morbidity, and mortality; hence, targeting EB's inflammatory footprint has emerged as the therapeutic goal [39]. EB Treatment is primarily supportive, focusing on wound care and symptom relief [39] (Figure9).
Figure 9: An illustration of the compromised basement membrane at the Dermo-Epidermal Junction seen in epidermolysis bullosa.
Investigated clones of primary epidermal stem cells obtained from skin biopsies from an EB patient corrected with a retrovirus. They applied them to the legs of 9 EB patients [18]. One year after the application, it was seen that the levels of laminin-5 synthesis in the 9 subjects were similar to a normal epidermis [18]. At the end of 6.5 years, no disease was observed in the transplanted areas, and a healthy epidermis had formed [18]. Displayed that 3 sessions of intravenous administrations of allogeneic umbilical cord blood MSCs [UC-MSCs] periodically on 6 patients with moderate-to-severe or severe RDEB were well tolerated and provided clinical benefits by reducing disease severity, disease-affected body area, blister count, and pain and itch and improving QOL on follow-up 5 months later [38].
Evaluated the administration of 3 cyclical intravenous injections of allogeneic BM-MSCs in 10 EB patients [31]. They reported that after 180 days of follow-up, the patient's quality of life increased and pain decreased; however, skin biopsies revealed no increase in type VII collagen expression nor new anchoring fibrils formation [31]. Epidermal stem cells with clonogenicity and long-term, lasting properties can permanently treat EB [18]. Clinical trials on EB patients conducted by Ebens et al. and Rashidghamat et al. using intravenously administered human BM-MSCs revealed stable to improved type VII collagen protein expression and gain of anchoring fibril components in skin biopsies and total blister count over the entire body surface area showed a decrease compared with baseline after a year follow-up [31]. Noted that transgenic MSCs could restore an entirely functional epidermis in a 7-year-old patient suffering from Junctional EB without any adverse events after 21 months of follow-up [22].
Researchers have generated an entire human epidermis using transgenic stem cells [25]. These long-lived stem cells ('holoclones') regenerated a stable, self-renewing epidermis without any visible scarring in the transplanted areas of the kin of a young patient with EB [25]. Interestingly, upon transplanting transgenic stem cells from an allogenic source, most of the cells from the graft disappeared after a few months [25]. Still, a small population of holoclone could regenerate multiple colonies for complete epidermis regeneration without any scars [25]. Further assessment in individuals with different forms of EB (EBS, JEB and DEB) showed more rapid healing in most subjects than standard dressings, with additional benefits in reducing pain and improving life quality [39]. A bi-layered allogeneic skin construct derived from the neonatal foreskin was used in treating a newborn with severe EBS, where the baby experienced good wound healing and accelerated the synthesis of trauma-resistant skin [39].
3D Vitiligo
Vitiligo is a disfiguring autoimmune disorder characterised by depigmented skin patches caused by deficiency or dysfunction in melanocytes that arise as a consequence of various genetic and external factors [22], [36]. The disease pathology ensures a progressive loss of skin pigmentation due to a gradual decrease or disappearance of melanocytes [22]. Poor progress in overcoming vitiligo is mainly down to a limited understanding of the mechanisms underlying the activation of melanocyte stem cells and their proliferation and migration [22]. Environmental factors, such as UV light, accelerate the generation of intracellular reactive oxygen species (ROS) and hydrogen peroxide and the initiation of melanogenesis, which liberates hydrogen peroxide [36]. Cellular stress induces ROS and activates the unfolded protein response (UPR), exacerbating vitiligo [36]. This response leads to the production of inflammatory cytokines (interleukin (IL)-6 and IL-8) by melanocytes, which can initiate a signalling cascade and antagonise the suppressive function of regulatory T cells [36]. The most practised and effective treatments include exposure to UV light and topical corticosteroids or vitamin D analogues [22]. These treatment modalities offer a temporary and incomplete remedy [22].
Interest in vitiligo research has recently focused on skin stem cells, mainly stem cells residing in the hair follicle, due to their potential in therapies to improve pigmentation [22]. eported that the co-administration of AD-MSCs with melanocytes derived from hair follicles was a safe and effective treatment modality for stable localised vitiligo [37]. The pigmentation of hair appeared preserved even in the skin areas affected by vitiligo, which denotes the presence of a reserve of melanocytes attributable to the structures of the hair follicle in the compromised areas [22]. A perifollicular distribution of the pigmentation is noticeable after exposure to UV irradiation [22]. This fact indicates an essential involvement of the precursors of the melanocytes residing in the hair follicle [22]. Melanocytic stem cells represent a promising object of study for new research aimed at clarifying their role in repigmentation and developing therapeutic strategies capable of effectively modulating their differentiation and consequent migration [22]. The Iranian Food and Drug Administration (FDA) authorised a live cell-based product containing a suspension of keratinocytes and melanocytes injected intraepidermal into the depigmented area for vitiligo [37].
Stem Cells in Aesthetic Medicine
4a. Photoaging
Ageing manifests architectural alteration and the functional decline of organs in an organism [21]. Ageing is the consequence of the irreversible deterioration of the organisms' physiological processes over time, and it is characterised by 9 hallmarks: cellular senescence, mitochondrial dysfunction, deregulated nutrient sensing, epigenetic alterations, telomere attrition, genomic instability, altered intercellular communication, and stem cell exhaustion [13]. Cellular senescence is a pathological process stirred up by the concomitant effects of extrinsic factors combined with intrinsic factors, such as a decline in DNA repair ability, increased oxidative stress, and telomeres' shortening [6]. Senescence is the end fate of cells when the regular cell cycle is arrested in the G1 phase, and there is the release of senescence-associated secretory phenotype (SASP), which modifies the microenvironment around senescent cells [13]. SASP contributes to the inflammatory reaction of ageing, a term describe as an asymptomatic chronic systemic low-grade, controlled inflammation associated with the ageing processes [13] (Figure 10).
Figure 10: An illustration of the 9 hallmarks of ageing.
Aged skin results from tissue loss of function and structural degeneration [12]. The skin undergoes matrix degradation, with a loss in collagen content, derangement of elastic fibres, increased oxidation activity, and the production of matrix metalloproteases (MMP) [12].
Skin morphology illustrates observable time passing by epidermal atrophy and fragility, leading to wrinkles, diminished dermal thickness and content, and pigmentary defects [6, 20]. Intrinsic factors associated with cell senescence impair the skin's physiological functions, including DNA damage, telomeres shortening, and reactive oxygen species (ROS) production [6]. All these processes play major roles in inducing tissue ageing [6]. Intrinsic ageing factors and structural and proliferative deficiencies bring on extrinsic ageing [6]. The decline in the number of epithelial cells, including DF, Langerhans cells, and melanocytes, combined with ECM degradation, results in an impairment of skin integrity and youthful guise [6]. Ageing skin loses its thickness, rete ridges, melanocytes and functional dermal fibroblasts [20]. Cutaneous fragility has been partly attributed to changes in hemidesmosomes, structures that connect keratinocytes to the basement membrane [20]. Epidermal cells and DF and their interaction with each other are critical in defining skin architecture and function [6]. The ECM proteins, glycosaminoglycans, collagen type I, type III, and elastin are mainly produced by DF and adipose stem cells and are continuously modified by physiological and extrinsic factors [6]. Collagen synthesis deteriorates simultaneously with its degradation, leading to quantitative and qualitative changes in collagen fibres' resting structure [6]. Collagen I and III are degraded by MMP-1, a ubiquitous endopeptidase secreted by keratinocytes, fibroblast and endothelial cells, while elastin degradation in the dermis is induced by MMP-2, -3, -9, and -12 [6]. MMP levels increase with age and are modulated by MMP tissue inhibitors (TIMPs) [6].
External physiological factors influencing photoaging include ultraviolet (UV) light, which breaks down ECM, precipitating a cascade of enzymatic activity leading to collagen degradation and loss of mechanical functions such as skin elasticity [6]. This insult to the skin physiologically predisposes it to carcinogenesis, besides cellular senescence [6] (Figure 11).
Figure 11: An illustration of a comparison of the histology between young and aged skin.
MSCs secrete TGF-β and IL-1β for macrophage recruitment and its polarisation from M1 to M2, followed by the secretion phase of anti-inflammatory cytokines to prevent the ageing process [6]. MSCs increase and improve dermal thickness by stimulating DF and angiogenesis to reduce wrinkle formation [6]. When administrated intradermally to aged skin, skin texture, wrinkles, and dermal thickness improve within 2 months of treatment [6]. MSCs from younger donors exhibited higher proliferation and regeneration capacity [6].
The mechanisms of stem cells in anti-ageing therapy are as follows [33]:
-
Regeneration and repair [33]
Stem cells proliferate and differentiate into various functional cells to restore ageing and damaged cells, maintaining integrated structures and the normal function of tissues and organs [33]
-
Microenvironment regulation [33]
Stem cells can secrete proteins, including growth factors and cytokines, and sustain cellular functions of tissues and organs through an intercellular signal transduction system, e.g. MSCs secrete endothelial growth factors, initiating microvascular endothelial progenitor cell proliferation and microvascular regeneration [33] (Figure 12).
Figure 12: An illustration of the clinical manifestation of ageing.
MSCs possess the mastery of nursing degenerative diseases as they have differentiation potential, replication capacity, and low immunogenicity [34]. They offer efficient re-epithelisation and growth factors necessary for skin regeneration in their anti-ageing armament [12]. MSC treatment increases elastic fibres in the dermis and modifies collagen and reticular fibre networks, hence repairing solar elastosis in photoaged skin [12]. The transplantation of MSCs leads to the complete renewal of dermal elastic matrix components, including xylan, melanin, and elastin fibrillary networks [12].
Noted that amniotic membrane-derived mesenchymal stem cells (AM-MSCs) administered via micro-needling to treat facial photoaging brought significant clinical improvement to photoaging, including diminishing pores, wrinkles, sun spots, and skin tone [12]. AM-MSCs improved the efficiency of DF migration and keratinocyte proliferation and increased collagen synthesis [12]. UCB-MSCs are renowned for their rapid cellular proliferation and immunomodulatory capacity [12]. Proved that UCB-MSCs contained growth factors such as EGF, FGF, TGF-β, PDGF, collagen type 1, hepatocyte growth factor (HGF), and a rejuvenation factor called growth differentiation factor 11 (GDF-11) [13]. They unveiled that a topical cream-based UCB-MSC applied daily on the skin for a month improved skin density by 2.46% and decreased eye wrinkles [12]. A retrospective study assessed the efficacy of a stromal vascular fraction (SVF) gel containing MSCs, growth factors, and extracellular matrix components for skin rejuvenation and volume augmentation on 126 subjects [23].
The SVF gel stimulated durable skin with enhanced volume augmentation and corrected dark lower eyelids and wrinkles, gradually lightening the skin [23]. Demonstrated that a cocktail containing MSCs from the skin and placenta combined with collagen and elastin administered intradermally to the face of 6 middle-aged female subjects weekly for 12 weeks improved the appearance of the subjects [42]. Analysis of dermal elastic filaments confirmed the reduction of skin roughness and the biosynthesis of new collagen and elastic fibres [42]. Clinical ameliorated features include skin hydration, senile lentigo quantity and uneven tone and pigmentation [42]. Reported that containing MSCs from the skin and placenta combined with pro-collagen peptides blended with hyaluronic acid injected subcutaneously weekly to the dorsum surface of the hands of 10 adult males over 10 weeks displayed an improvement to the hydration, skin thickness and elasticity of the skin [43].
4B Scars
Scar formation is a naturally occurring spontaneous healing procedure after trauma, injury, infection, and surgery to anybody [25]. Scarring impacts self-esteem, bringing seclusion, sentiments of defenselessness, and low social confidence to the bearer [25]. Generally, a scar is a biological reaction triggered by skin damage and underlying tissue [25]. Scars can be clinically classified as hydrotropic, normotrophic, contracture, hypertrophic and keloid scars, which occur due to collagen fibrous tissue overgrowth that replaces normal skin during healing [25]. Hypertrophic scars are raised and oddly pigmented, comprised of myofibroblasts, collagen fibres, and extracellular matrix (ECM) [25]. Compared with keloid scars, hypertrophic scars stay within the limit of the area of initial damage and relapse with time [25].
Published that DF is essential for the scarless healing population as they synthesise ECM components, such as fibronectin and tenascin [25]. The profibrotic growth factors, TGFβ1 and platelet-derived growth factor (PDGF), are essential for promoting myofibroblast activation at wound healing sites [25]. Matrix metalloproteinases (MMP) and keratins, such as K8 and K1925, support ECM remodelling and wound healing. Uncovered that growth factors, such as basic fibroblast growth factor (bFGF) and PDGF, stimulated the proliferation of DF [25]. Reported that transdermal injection of allogeneic UC-MSCs of varying concentrations of stem cells on 90 patients with hypertrophic scars from caesarean section revealed no apparent adverse effects. Efficacy was evaluated using the Vancouver Scar Scale (VSS), demonstrating that after 6 months, the high-dose group fared better against the low-dose group, respectively [31]. MSC-Exosomes avert fibroblasts to myofibroblasts conversion and inhibit granular tissue formation by increasing the TGF-β3: TGF-β1 ratio [25]. MSC-Exosomes promote collagen remodelling in a scarless pattern, enhancing angiogenesis to reduce scar formation probability [25]. Published that MSC-Exosomes contain specific miRNAs like miR-21, miR-23a, miR-125b, and miR-145) that accelerate full-thickness skin wound healing and attenuate scar formation by stimulating fibroblast proliferation and migration, including collagen deposition, via activation of the PI3K/Akt signalling pathway and play an essential role in suppressing myofibroblast formation by inhibiting the TGFβ2/SMAD2 pathway, which inhibits collagen expression to reduce scar formation during the later stage of wound healing [25].
4C Hair Loss
Hair contributes to a healthy life through sensory effects, thermoregulation, physical protection, and, most importantly, social impacts [24]. Androgenic alopecia (AGA) is a ponderous patterned hair loss in both men and women [12]. AGA is the most common cause of hair loss, affecting 30–50% of men (male-pattern hair loss [MPHL]) and 30% of middle-aged women (female-pattern hair loss [FPHL]) [40]. AGA is characterised by a pathologic hair follicle miniaturisation caused by perturbation of the growth cycle via dihydrotestosterone (DHT) accumulation [40].
DHT accumulates due to testosterone metabolism inhibition by 5α-reductase [40]. DHT binds to the hair follicle's androgen receptor, lowering cyclic AMP (cAMP) concentration, reducing sugar metabolism and suppressing the energy supply required for hair follicle growth [12]. Current treatment modalities include oral medications such as Finasteride®, topicals such as Minoxidil, and surgical procedures such as hair transplantation [40]. In alopecic scalps, the hair follicle stem cell numbers remain unaltered; however, the quantity of the more effectively multiplying progenitor cells significantly decreases [40]. Several studies have shown that human hair follicle stem cells (HFSC) and dermal papilla cells (DPC) hamper hair loss [22]. The stem cells furnish hair follicles with optimal conditions for hair regeneration [22]. The role of stem cells in hair follicle regeneration involves the Wnt/β-catenin cycle programming [12].
Investigated the interactions between DPCs and MSCs in increasing hair formation [12]. Mesenchyme signals derived from stem cells and growth factors influence hair growth through cell proliferation to prolong the anagen phase, induce cell growth (ERK activation), stimulate hair follicle development (β-catenin) and suppress apoptotic signals (release of Bcl-2 and activation of Akt) [22]. Reported that sub-cutaneous scalp injections of fat tissue in patients affected by AGA augmented an average of 31.0 hairs/cm2 growth [40]. Achieved similar results on 22 patients using AD-MSCs [40]. Demonstrated that administering AD-MSCs to 27 women with FPHL over 12 weeks improved hair thickness and density without serious side effects [40]. Reported that MSCs-Exos stimulated the proliferation and migration of DPCs and induced the secretion of VEGF and IGF-1 [12, 13].
Safety Issue of MSC Therapy
Stem cell therapy has been relatively safe mainly due to the high standards and precautions from initial harvesting to transplantation. The type of stem cells utilised, their route of administration, and their ability to differentiate and proliferate in the host system all account for safe transplantation [3]. Risk factors are generally classified as intrinsic factors: cell lifespan, viability, proliferative capacity, differentiation status, and tumorigenic potential, and extrinsic factors: handling protocols, contaminants, transport, etc. and storage conditions [3]. Clinical safety in stem cell application starts during cell culturing and handling of cell lines, as the purity and identity of the cells play a crucial role in the safety and efficacy of the treatment [3]. The controversial concern in stem cell therapy is the risk of tumour formation [3]. Another is contamination by bacteria, viruses, fungi, or prion pathogens, which leads to disease transmission to the recipient [3]. Other risk factors include adverse exaggerated immune response and the communication of adventitious agents [3].
MSCs demonstrate an excellent potential to differentiate into multiple tissues; therefore, they risk unwanted differentiation of transplanted MSCs, which may promote tumour growth [32]. Furthermore, it is well-accepted that angiogenesis plays a vital role in tumour invasion and metastasis of tumours [32]. MSCs differentiate into vascular endothelial cells, secreting several growth factors, including VEGF and PDGF, which promote tumour angiogenesis and invasive behaviour [32]. MSCs also play a role in tumour invasion and metastasis, known as epithelial to mesenchymal transition (EMT), which drives tumour cells to lose polarity and acquire invasive phenotype [32]. A large quantity of stem cells is sometimes injected to obtain a potentially higher beneficial effect from stem cell therapy; paradoxically, this leads to a higher risk of unwanted effects [3]. When injected through a small needle, more cells aggregate to bring forth infarction [3]. Following administration, stem cells release specific soluble mediators, which can modulate the immune system's effector function, leading to immunosuppressive and anti-inflammatory effects [3]. In general, the tumorigenic potential of stem cells is induced by the local environment [3].
Conclusion
Mesenchymal stem cell therapy is one of the most potent and exciting options for regenerative therapies. It has shown the potential to regenerate or rejuvenate impaired tissue in a clinical setting. MSCs exert their therapeutic effects through secretion-based paracrine mechanisms rather than direct cellular replacement. The discovery of Induced Pluripotent Stem Cell-Derived MSCs (iMSCs) from controlled differentiation of iPSCs to obtain a homogeneous population represents a promising alternative for regenerative therapy.
References
1. Fu X, Liu G, Halim A, Ju Y, Luo Q, et al. (2019) Mesenchymal Stem Cell Migration and Tissue Repair. Cells. 8(8):784.
2. Hade MD, Suire CN, Suo Z. (2021) Mesenchymal Stem Cell-Derived Exosomes: Applications in Regenerative Medicine. Cells. 10(8):1959.
3. Das M, Mayilsamy K, Mohapatra SS, Mohapatra S. (2019) Mesenchymal stem cell therapy for treating traumatic brain injury: progress and prospects. Rev Neurosci. 30(8):839-55.
4. Lan T, Luo M, Wei X. (2018) Mesenchymal stem/stromal cells in cancer therapy. J Hematol Oncol. 2021;14(1):195.
5. Galipeau J, Sensébé L. Mesenchymal Stromal Cells: Clinical Challenges and Therapeutic Opportunities. Cell Stem Cell. 22(6):824-33.
6. Mazini L, Rochette L, Admou B, Amal S, Malka G. (2020) Hopes and Limits of Adipose-Derived Stem Cells (ADSCs) and Mesenchymal Stem Cells (MSCs) in Wound Healing. Int J Mol Sci. 21(4):1306.
7. Naji A, Eitoku M, Favier B, Deschaseaux F, Rouas-Freiss N, et al. (2019) Suganuma N. Biological functions of mesenchymal stem cells and clinical implications. Cell Mol Life Sci. 2019;76(17):3323-48.
8. Slukvin II, Kumar A. (2018) The mesenchymoangioblast is a mesodermal precursor for mesenchymal and endothelial cells. Cell Mol Life Sci. 75(19):3507-20.
9. Cao J, Spielmann M, Qiu X, Huang X, Ibrahim DM, (2019) The single-cell transcriptional landscape of mammalian organogenesis. Nature. 566(7745):496-502.
10. McGrath M, Tam E, Sladkova M, AlManaie A, Zimmer M, et al. (2019) GMP-compatible and xeno-free cultivation of mesenchymal progenitors derived from human induced pluripotent stem cells. Stem Cell Res Ther. 10(1):11.
11. An Y, Lin S, Tan X, Zhu S, Nie F, et al. (2021) Exosomes from adipose-derived stem cells and application to skin wound healing. Cell Prolif. 54(3):e12993.
12. Jo H, Brito S, Kwak BM, Park S, Lee MG, et al. (2021) Applications of Mesenchymal Stem Cells in Skin Regeneration and Rejuvenation. Int J Mol Sci. 22(5):2410.
13. Ha DH, Kim HK, Lee J, Kwon HH, Park GH, et al. (2020) Mesenchymal Stem/Stromal Cell-Derived Exosomes for Immunomodulatory Therapeutics and Skin Regeneration. Cells. 9(5):1157.
14. Li KN, Tumbar T. (2021) Hair follicle stem cells as a skin-organising signalling centre during adult homeostasis. EMBO J. 40(11):e107135
15. Taub AF, Pham K. (2018) Stem Cells in Dermatology and Anti-aging Care of the Skin. Facial Plast Surg Clin North Am. 26(4):425-37.
16. Hassanshahi A, Hassanshahi M, Khabbazi S, Hosseini-Khah Z, Peymanfar Y, et al. (2019) Adipose-derived stem cells for wound healing. J Cell Physiol. 234(6):7903-14.
17. Nourian Dehkordi A, Mirahmadi Babaheydari F, Chehelgerdi M, Raeisi Dehkordi S. (2019) Skin tissue engineering: wound healing based on stem-cell-based therapeutic strategies. Stem Cell Res Ther. 10(1):111
18. Çankirili NK, Altundag O, Çelebi-Saltik B. (2020) Skin Stem Cells, Their Niche and Tissue Engineering Approach for Skin Regeneration. Adv Exp Med Biol. 1212:107-126
19. Díaz-García D, Filipová A, Garza-Veloz I, Martinez-Fierro ML. (2021) A Beginner's Introduction to Skin Stem Cells and Wound Healing. Int J Mol Sci. 22(20):11030.
20. Liu N, Matsumura H, Kato T, Ichinose S, Takada A, et al. (2019) Stem cell competition orchestrates skin homeostasis and ageing. Nature. 2019;568(7752):344-50.
21. Ge Y, Miao Y, Gur-Cohen S, Gomez N, Yang H, et al. (2020) The ageing skin microenvironment dictates stem cell behaviour. Proc Natl Acad Sci U S A. 117(10):5339-50.
22. Ahmadi-Ashtiani HR, Bishe P, Baldisserotto A, Buso P, Manfredini S, et al. (2020) Stem Cells as a Target for the Delivery of Active Molecules to Skin by Topical Administration. Int J Mol Sci. 21(6):2251
23. Wang JV, Schoenberg E, Zaya R, Rohrer T, Zachary CB, et al. (2020) The rise of stem cells in skin rejuvenation: A new frontier. Clin Dermatol. 38(4):494-6.
24. Hu XM, Li ZX, Zhang DY, Yang YC, Fu SA,et al. (2021) A systematic summary of survival and death signalling during the life of hair follicle stem cells. Stem Cell Res Ther. 12(1):453
25. Giri S, Machens HG, Bader A. (2019) Therapeutic potential of endogenous stem cells and cellular factors for scar-free skin regeneration. Drug Discov Today. 24(1):69-84.
26. Schneider I, Calcagni M, Buschmann J. (2023) Adipose-derived stem cells applied in skin diseases, wound healing and skin defects: a review. Cytotherapy. 25(2):105-119.
27. Mahmoudian-Sani MR, Rafeei F, Amini R, Saidijam M. (2018) The effect of mesenchymal stem cells combined with platelet-rich plasma on skin wound healing. J Cosmet Dermatol. 17(5):650-9.
28. Yang J, Xiao M, Ma K, Li H, Ran M, et al. (2023) Therapeutic effects of mesenchymal stem cells and their derivatives in common skin inflammatory diseases: Atopic dermatitis and psoriasis. Front Immunol. 14:1092668.
29. Daltro SRT, Meira CS, Santos IP, Ribeiro Dos Santos R, Soares MBP. (2020) Mesenchymal Stem Cells and Atopic Dermatitis: A Review. Front Cell Dev Biol. 8:326.
30. Hua C, Chen S, Cheng H. (2022) Therapeutic potential of mesenchymal stem cells for refractory inflammatory and immune skin diseases. Hum Vaccin Immunother. 18(6):2144667.
31. Sierra-Sánchez Á, Montero-Vilchez T, Quiñones-Vico MI, Sanchez-Diaz M, Arias-Santiago S. (2021) Current Advanced Therapies Based on Human Mesenchymal Stem Cells for Skin Diseases. Front Cell Dev Biol. 9:643125.
32. Zhu Y, Ge J, Huang C, Liu H, Jiang H. (2021) Application of mesenchymal stem cell therapy for ageing frailty: from mechanisms to therapeutics. Theranostics. 11(12):5675-85.
33. Yu Y. (2018) Application of Stem Cell Technology in Anti-aging and Aging-Related Diseases. Adv Exp Med Biol. 1086:255-65.
34. Chen H, Liu O, Chen S, Zhou Y. (2022) Aging and Mesenchymal Stem Cells: Therapeutic Opportunities and Challenges in the Older Group. Gerontology. 68(3):339-52.
35. Domaszewska-Szostek A, Krzyżanowska M, Siemionow M. (2019) Cell-Based Therapies for Chronic Wounds Tested in Clinical Studies: Review. Ann Plast Surg. 83(6):e96-e109.
36. Zhu L, Lin X, Zhi L, Fang Y, Lin K, et al. (2020) Mesenchymal stem cells promote human melanocytes proliferation and resistance to apoptosis through PTEN pathway in vitiligo. Stem Cell Res Ther. 11(1):26.
37. Ghashghaei S, Abbaszadeh M, Karimi S, Ataie-Fashtami L, et al. (2023) Advanced Therapy Medicinal Products in Vitiligo; Current Status, Future Prospect, and Approved Treatments. Cell J. 25(3):143-57.
38. Lee SE, Lee SJ, Kim SE, Kim K, Cho B, et al. (2021) Intravenous allogeneic umbilical cord blood-derived mesenchymal stem cell therapy in recessive dystrophic epidermolysis bullosa patients. JCI Insight. 6(2):e143606.
39. Hou PC, Wang HT, Abhee S, Tu WT, McGrath JA, et al. (2021) Investigational Treatments for Epidermolysis Bullosa. Am J Clin Dermatol. 22(6):801-17.
40. Shimizu Y, Ntege EH, Sunami H, Inoue Y. (2022) Regenerative medicine strategies for hair growth and regeneration: A narrative literature review. Regen Ther. 21:527-39.
41. Ye C, Zhang Y, Su Z, Wu S, Li Y, et al. (2022) hMSC exosomes as a novel treatment for female sensitive skin: An in vivo study. Front Bioeng Biotechnol. 10:1053679.
42. Klokol D, Tulina D, Teppone M, Chan M, Wong M, et al. (2016) Application of Cell Extracts from Skin, Placenta, Mesenchyme with Collagen and Elastin in Aesthetic Dermatology and Skin Revitalization: Evaluation of Outcomes in Cohort Study. Am J Adv Drug Del. 5(4):69-77
43. Chernykh V, Nallenthiran L, Yemeliyanova M. (2020) Novel Technique of Aesthetic Skin Rejuvenation with Cellular Extracted Compounds Enriched with Tissue-Specific Peptides and Hyaluronic Acid. J Stem Cell Res Dev Ther. 6:32.
This article was originally published in a special issue entitled “Precision Cell Therapy”, handled by Editor Dr. Roni Moya.